Sickle Cell Hemoglobin mRNA Sequence Analysis
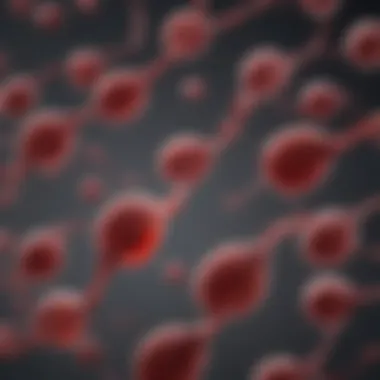
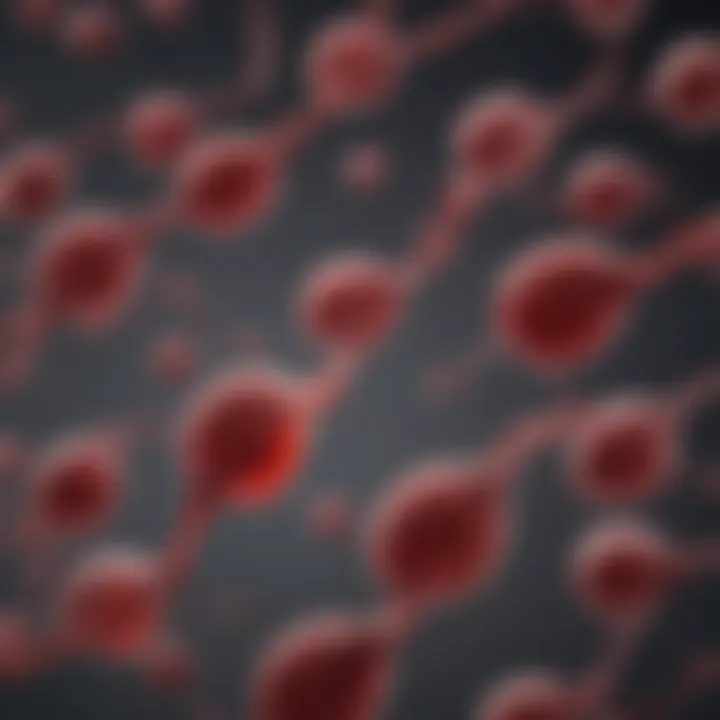
Intro
Sickle cell disease is not merely a blood disorder; it's a window into the complex world of genetics and molecular biology. At the heart of this condition lies a single mutation in the mRNA sequence that leads to the production of an abnormal hemoglobin, known as hemoglobin S. The implications of this molecular alteration stretch far beyond the individual level, affecting families, communities, and healthcare systems alike. To grasp the full extent of sickle cell disease, an exploration of the mRNA sequence responsible for this abnormality is essential. It opens a door to understanding how genetic mutations can manifest into significant health issues, providing clues that could lead to innovative therapeutic strategies.
Research Overview
Summary of Key Findings
Recent studies have demonstrated that a slight change in the mRNA sequence can have tremendous effects on the functionality of hemoglobin. This mutation can lead to sickling of red blood cells, causing them to lose their flexibility and obstruct blood flow. Notably, the findings suggest that peeling back the layers of sickle cell hemoglobin mRNA can provide insights into potential gene therapies. Enhancements in sequencing technologies have also allowed researchers to identify variations in mRNA sequences, paving pathways toward personalized medicine approaches.
Background and Context
Understanding sickle cell disease necessitates a foundation in hemoglobin structure and function. Normal hemoglobin, a crucial protein that carries oxygen in blood, is composed of two types of globin chains: alpha and beta. The mRNA sequence encoding hemoglobin is not just a series of nucleotides; it's a code that orchestrates protein synthesis. The aberration that leads to sickle cell disease occurs in the beta-globin gene on chromosome 11, where a single nucleotide change from A to T results in the substitution of valine for glutamic acid in the protein sequence. This seemingly trivial alteration is the root cause of various clinical manifestations associated with sickle cell disease, including pain crises, organ damage, and increased risk of infections.
Sickle cell disease has a distinct geographic prevalence, prominently affecting individuals of African descent, although it is also observed in Mediterranean, Middle Eastern, and Indian populations. This disease has been the focus of significant medical and genetic research, ultimately contributing to enhanced awareness and treatment guidelines.
"The key to unraveling the complexities of sickle cell disease is understanding its molecular foundation. This knowledge guides us toward better treatment options and deeper insights into genetic disorders."
— Expert in Hematology
In the sections to come, we will delve into the methodologies used in studying the mRNA sequence of sickle cell hemoglobin, as well as the innovative research that holds the potential for transformative therapies.
Prelims to Sickle Cell Hemoglobin
The exploration of sickle cell hemoglobin is not just a scientific venture; it's a quest to unravel the complexities of human health and disease. Understanding sickle cell hemoglobin—the variant associated with sickle cell disease—opens up a window into the wider mechanisms of genetics, protein synthesis, and the implications of cellular mutations. This discussion holds particular significance for folks in medicine and research, as each nuance in the mRNA sequence could hold keys to innovative treatments, predictive diagnostics, or even prevention strategies.
Sickle cell hemoglobin brings with it a host of challenges, from the pathophysiology underlying the disease to the overarching impact on patients’ lives. The unique characteristics of the altered hemoglobin influence not just the structure of red blood cells but also how these cells react under various physiological stresses. In short, examining the genesis of sickle cell hemoglobin equips practitioners and researchers alike with insights that are imperative for developing targeted therapies.
Defining Sickle Cell Disease
Sickle cell disease is a hereditary condition that primarily affects hemoglobin, the protein responsible for transporting oxygen in red blood cells. Unlike normal hemoglobin, where the beta-globin chain remains unchanged, the sickle variant results from a single nucleotide mutation in the HBB gene. This seemingly small alteration triggers a cascade of changes in the structure of hemoglobin, causing red blood cells to distort into a characteristic crescent or "sickle" shape.
This transformation is more than just a visual difference; it severely impacts the cells' functionality. The sickle cells tend to clump together, leading to blockage within blood vessels. As a consequence, patients face a myriad of complications ranging from chronic pain episodes, known as sickle cell crises, to increased risk of infections and stroke. The burden of this disease underscores the urgent need for research focused on the underlying genetics and mechanisms at play.
Historical Context and Discoveries
The journey towards understanding sickle cell disease has been paved with robust research and intriguing discoveries. It all started back in 1910 when Dr. James B. Herrick first described the peculiar sickle-shaped cells in a patient with severe anemia. This marked a pivotal moment in the medical community, igniting interest that would eventually lead to significant advances in genetic understanding.
In 1949, Linus Pauling made an important leap in defining sickle cell disease as a molecular disease, a term that described how a change in a single amino acid within the hemoglobin variant could yield drastic physiological outcomes. This laid the groundwork for modern genetic research, highlighting the relationship between genetics and disease phenotypes.
Since then, a wealth of research has evolved, making it abundantly clear that the implications of sickle cell hemoglobin extend far beyond just the individual. Global health initiatives have aimed to address the epidemic, particularly in endemic areas like sub-Saharan Africa. With constant advancements in genomics, understanding sickle cell disease from its molecular pathways to its impact on populations continues to be a vibrant field of study, ripe for exploration and discovery.
The Biochemistry of Hemoglobin
Understanding the biochemistry of hemoglobin is crucial to grasping the overall theme of this article. Hemoglobin not only carries oxygen from the lungs to the body's tissues but also facilitates the return transport of carbon dioxide from the tissues back to the lungs. This dual role is vital in ensuring that our body functions efficiently without any hitches. Moreover, the way hemoglobin interacts with oxygen and how any alterations affect its function are central to the discussions on sickle cell disease.
From a biochemical perspective, it’s not just about the molecules but their structural intricacies—how changes at the molecular level can lead to significant physiological consequences. It's here that nuances like pH, temperature, and other environmental factors come into play, impacting hemoglobin's affinity for oxygen. This interplay lays the groundwork for understanding the shifts from normal hemoglobin to its sickle variant.
Structure of Normal Hemoglobin
Normal hemoglobin, referred to as hemoglobin A, is a globular protein composed of four polypeptide chains: two alpha and two beta chains. Each of these chains contains an iron-containing heme group, which is responsible for the protein's oxygen-binding capacity. The quaternary structure of hemoglobin allows for cooperative binding; when one heme group binds to oxygen, the others adjust their conformation, increasing the likelihood that additional oxygen molecules will bind. This property is akin to a well-rehearsed musical performance, where each part contributes to a harmonious outcome.
The typical structure can be broken down as follows:
- Tertiary Structure: Individual chains fold into specific shapes that are critical for function.
- Quaternary Structure: The interaction between the four chains forms a functional unit capable of binding oxygen.
- Heme Groups: Iron at the center of the heme is what holds oxygen, forming a complex network that ensures efficient oxygen transport.
Understanding this structure highlights the complexity of hemoglobin's role and lays the foundation for comprehending how minor mutations can cause drastic changes in its functionality.
Alterations in Sickle Cell Hemoglobin
Sickle cell hemoglobin, often labeled as hemoglobin S, arises from a single nucleotide polymorphism in the beta-globin gene. This mutation leads to a substitution of valine for glutamic acid at the sixth position of the beta globin chain. Such a seemingly trivial alteration transforms the way hemoglobin behaves. While normal hemoglobin has a smooth, flexible structure, sickle cell hemoglobin does not.
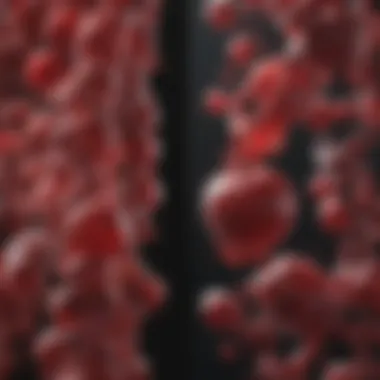
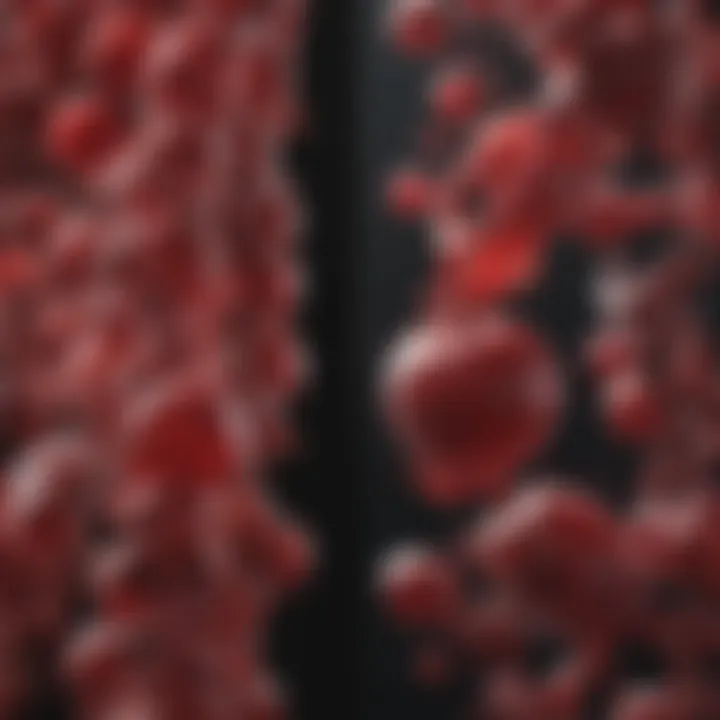
The consequences of these alterations are extensive:
- Shape Change: Sickle hemoglobin tends to polymerize under low oxygen conditions, causing red blood cells to assume a rigid, sickle-like shape. This not only blocks small blood vessels but also leads to reduced lifespan of the cells.
- Decreased Oxygen Transport: The structural change in hemoglobin S leads to decreased oxygen-carrying capacity, which can instigate symptoms like fatigue and pain crisis in patients.
- Increased Hemolysis: The abnormal shape of sickle cells leads to their premature destruction in the spleen, which can lead to anemia.
It’s no easy feat tackling the implications of these alterations. They ripple through the entire vascular system, affecting oxygen delivery and overall metabolic processes.
"In the sphere of biochemistry, small changes often carry monumental consequences; sickle cell hemoglobin is a living example of this truth."
The understanding of these biochemical pathways blends seamlessly into wider discussions on treatment options and genetic implications for those afflicted with sickle cell disease.
Genetic Basis of Sickle Cell Hemoglobin
Understanding the genetic foundation of sickle cell hemoglobin is crucial, as it lays the cornerstone for both the pathology of sickle cell disease and the path forward in treatment strategies. Sickle cell disease is not merely a manifestation of symptoms but is intricately woven into the genetic fabric of individuals. At the heart of this condition is a singular point mutation in the beta-globin gene, which encodes for one of the chains that form hemoglobin.
Mutation in the Hemoglobin Gene
The sickle cell mutation occurs specifically in the HBB gene located on chromosome 11. This gene normally codes for the beta subunit of hemoglobin. A single nucleotide change—where adenine is swapped for thymine—leads to the substitution of glutamic acid with valine in the second position of the beta-globin chain. This seemingly inconsequential alteration has profound consequences. The mutant form of hemoglobin, known as hemoglobin S (HbS), tends to polymerize under low oxygen conditions, leading to the distortion of red blood cells into a sickle shape.
These sickle-shaped cells can cause blockages in small blood vessels, reducing blood flow and leading to painful crises. It's these crises that often bring patients to medical attention, highlighting the urgent need for better understanding of the underlying genetics. Moreover, individuals who are carriers (those with one normal and one sickle allele) may have a protective advantage against malaria, revealing a complex interplay between genetics and evolutionary pressures.
Inheritance Patterns and Epidemiology
The inheritance of sickle cell disease follows an autosomal recessive pattern. This means that a person must inherit two copies of the mutated HBB gene, one from each parent, to express the disease. If only one copy is inherited, that individual is a carrier, often without symptoms but capable of passing the mutation to offspring.
The epidemiological aspects of sickle cell disease reflect its prevalence in specific geographic regions. Areas like Sub-Saharan Africa, the Mediterranean, and parts of India have higher frequencies of the sickle cell trait. This pattern is believed to be linked with the historical prevalence of malaria in these regions.
"The sickle cell mutation is not merely a genetic anomaly; rather, it exists at the crossroads of health, disease, and cultural identity."
In terms of public health, understanding these inheritance patterns is vital. Genetic counseling becomes increasingly important for families with histories of the disease, aiding in informed family planning. The rising interest in gene therapy and targeted treatments stems directly from comprehending these genetic foundations, suggesting future avenues for intervention.
As research continues, the implications of these genetic discoveries are vast. They pave the way for more personalized medicine approaches, and a greater understanding of both sickle cell disease and its relationship to other hemoglobinopathies. By addressing these genetic concerns, healthcare systems can better target prevention strategies and improve outcomes for those affected.
mRNA Sequence of Sickle Cell Hemoglobin
The examination of the mRNA sequence of sickle cell hemoglobin stands as a cornerstone for understanding both the pathological and therapeutic landscapes of sickle cell disease. Investigating this sequence sheds light on the specific genetic alterations that lead to the formation of an abnormal hemoglobin variant, ultimately impacting patients' health. Dissecting the mRNA offers insights not just into the molecular mechanism of disease, but also uncovers potential pathways for intervention.
One of the crucial elements to consider is the transcriptional process itself. It lays the groundwork for protein synthesis, enabling researchers to grasp how slight variations in the mRNA can drastically shift the biological landscape in affected individuals. Furthermore, understanding the precise distinctions between mutant and wild-type mRNA sequences provides a crucial framework for developing targeted therapies.
Collectively, examining mRNA sequences also opens the door to advanced sequencing technologies—changing the way we gather genetic information and changing treatment paradigms as well. Considering these implications is essential, as this knowledge forms the bedrock upon which future research can build.
Transcriptional Dynamics of the β-globin Gene
Transcription dynamics of the β-globin gene tie directly to the synthesis of hemoglobin. Initially, transcription starts when RNA polymerase binds to a promoter region on the DNA, leading to the unwinding of the double helix. A number of factors affect this dynamic process—environmental conditions, cellular signals, and regulatory proteins all play pivotal roles.
Essentially, upon the initiation of transcription, pre-mRNA is synthesized from the template DNA strand. This pre-mRNA undergoes splicing and modifications to mature into functional mRNA. In sickle cell disease, the transcription of the β-globin gene does not occur in a vacuum. Factors such as transcription factor availability and chromatin accessibility can greatly influence how well the gene expresses, with misregulation contributing to the production of dysfunctional mRNA.
Moreover, the dynamics of transcription must incorporate the phenomenon of alternative splicing, where different combinations of exons can lead to varied mRNA outputs. This creates a diverse range of possible protein derivatives. Understanding these dynamics helps in unraveling the complexities behind how an abnormal hemoglobin variant arises.
Mutant versus Wild-Type mRNA Sequences
When comparing mutant and wild-type mRNA sequences, the differences become stark and significant. In sickle cell disease, a single nucleotide change in the β-globin gene leads to the substitution of valine for glutamic acid at the sixth position. This seemingly minor alteration creates a ripple effect, ultimately culminating in the drastic changes observed in both structure and function of hemoglobin.
To better illustrate this:
- Wild-Type mRNA Sequence: Typically produces functional β-globin, allowing normal oxygen transport and solubility in blood plasma.
- Mutant mRNA Sequence: Produces sickle hemoglobin (HbS), which can become rigid and polymerize under low oxygen conditions, leading to the sickling of red blood cells.
The implications of these mRNA variations are profound. The abnormal mRNA sequence not only alters protein folding but also detrimentally affects oxygen delivery throughout the body. This newly shaped hemoglobin does not just cause the red blood cells to deform; it initiates a cascade of pathophysiological events that characterize sickle cell disease.
Understanding these variations is paramount for developing treatments aimed at correcting or mitigating these mutations. Whether it’s through gene therapy, CRISPR technology, or targeted small molecules, a thorough comprehension of mutant versus wild-type mRNA sequences paves the way toward innovative and more efficacious treatment options.
Implications of mRNA Sequence Variations
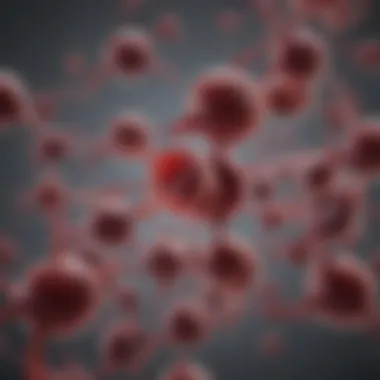

The exploration of mRNA sequence variations holds significant weight in understanding sickle cell hemoglobin. These variations, arising from substitutions in the mRNA code, can lead to notable differences in the resulting protein structure and function, thus affecting the overall health of individuals with sickle cell disease. This section digs into the consequences of such variations, emphasizing specific elements that highlight their importance, including how changes in mRNA can alter folding, functionality, and ultimately impact therapeutic approaches.
Effects on Protein Folding and Functionality
One of the most critical aspects of mRNA sequence variations is their impact on protein folding. Normal hemoglobin, comprised of two alpha and two beta chains, relies on a precise sequence to fold properly into a functional molecule. In sickle cell hemoglobin, the substitution of adenine with thymine in the mRNA sequence impacts the beta-globin chain. This seemingly minor change transforms the protein's structure into a form that favors aggregation under low-oxygen conditions.
- Unraveling Protein Stability: The misfolded beta-globin chains tend to stick together, forming polymer strands that distort red blood cells into a characteristic sickle shape. This condition can lead to complications like vaso-occlusive crises, an excruciating blockage in blood flow.
- Impaired Functionality: Sickle hemoglobin's inability to efficiently transport oxygen can result in both chronic fatigue and acute episodes of pain, significantly hampering the quality of life for affected individuals.
Understanding these protein folding nuances offers valuable insight for researchers. By targeting the specific sequence variations in mRNA, novel therapeutic interventions might be developed to encourage proper folding and restore normal functionality.
Pathophysiological Consequences of Sickle Cell Mutation
The implications of mRNA sequence variations extend beyond structural changes. These alterations can have a cascade of pathophysiological effects that impact the broader health landscape for individuals with sickle cell disease.
- Increased Hemolysis: Sickle cells are more fragile and prone to breaking apart, leading to hemolytic anemia where the body does not have enough red blood cells. This can cause fatigue, weakness, and complications from iron overload.
- Organ Damage: Repeated sickling and unsickling of red blood cells can cause micro-infarctions in various organs. These micro-infarcts can accumulate over time, resulting in long-term damage and functional impairments in organs like the spleen, kidneys, or even the heart.
- Pain Crises: As mentioned earlier, the blockages caused by sickle-shaped cells lead to painful episodes known as "crises," which contribute to the disease's burden and significantly affect the wellbeing of patients.
Technological Advances in mRNA Sequencing
The landscape of molecular biology has transformed significantly within the last couple of decades, especially concerning the way we understand genetic information. One of the most pivotal shifts has been the advancement of mRNA sequencing technology. Understanding how mRNA serves as a blueprint for protein synthesis offers insights into diseases like sickle cell anemia. Improved sequencing technologies provide a more nuanced understanding of the mRNA structure, which ultimately enhances our grasp of the underlying genetic disorders.
These technological advancements have not only helped unravel the complexities associated with sickle cell hemoglobin but also opened doors to new therapeutic possibilities. Here are some key elements that highlight the importance of modern mRNA sequencing:
- Precision: Next-generation sequencing allows for a level of refinement that is simply unattainable with earlier methods. This means scientists can pinpoint mutations in the sickle cell mRNA with a degree of accuracy that aids in both diagnosis and treatment.
- Speed: With current sequencing techniques, researchers can process large volumes of data rapidly. This efficiency is essential when time can be critical in clinical settings.
- Affordability: As the technology has progressed, the cost of sequencing has decreased. This democratizes access to genetic testing and can lead to broader screening programs in communities disproportionately affected by sickle cell disease.
"The evolution of sequencing technology is akin to upgrading from a magnifying glass to a high-definition microscope. The details that were once obscured are now vividly clear."
These benefits underscore the vital role that technology plays in understanding mRNA sequences. More than just an academic exercise, these advancements have practical implications for patient care, especially for those suffering from genetic conditions. Moreover, as we utilize these technologies, it’s crucial to discuss the tools that help researchers decipher the extensive data generated through mRNA sequencing.
Next-Generation Sequencing Techniques
Next-generation sequencing, or NGS, is at the forefront of technological progress in molecular biology. NGS offers a dramatically enhanced capacity to analyze sequences compared to traditional methods like Sanger sequencing. When looking at sickle cell hemoglobin, NGS enables researchers to examine the variant mRNA sequences alongside normal ones, elucidating the precise changes that give rise to sickle cell disease.
The methodologies employed in NGS often encompass:
- Whole Transcriptome Sequencing: This method allows for the capture of all RNA molecules in a sample, providing comprehensive insight into gene expression levels, including those affected in sickle cell disease.
- Targeted Sequencing: By focusing on specific genes of interest—like the β-globin gene—researchers can funnel their resources and time into understanding significant mutations that contribute to hemoglobinopathies.
- Single-Cell Sequencing: This technique opens a new chapter in analyzing mRNA from individual cells, which can uncover variations in gene expression that might be masked in bulk samples, providing richer data on how sickle cell manifestations can differ from patient to patient.
Each technique brings forward its own set of advantages and challenges but collectively enhances our understanding of mRNA dynamics.
Bioinformatics Tools for Sequence Analysis
With advancements in sequencing technologies comes the need for robust bioinformatics tools. These programs are indispensable for managing the massive datasets that result from mRNA sequencing efforts. In particular, bioinformatics plays a critical role in analyzing the mRNA sequences associated with sickle cell hemoglobin.
Some vital bioinformatics tools include:
- BLAST (Basic Local Alignment Search Tool): This software helps researchers identify similarities between nucleotide sequences. By aligning sickle cell mRNA against reference sequences, one can detect mutations that alter protein function.
- STAR (Spliced Transcript Alignment to a Reference): This tool is particularly designed for aligning RNA-Seq reads. Its ability to handle splice variants makes it especially useful for studying mRNA sequences associated with sickle cell disease.
- GATK (Genome Analysis Toolkit): Originally developed for DNA sequencing, GATK has data processing capabilities that can be adapted for RNA sequencing, allowing for variant calls in different expressed sequences.
- DESeq2: This package is essential for analyzing differential gene expression data, which can highlight changes in mRNA levels between healthy and sickle cell patients.
Utilizing these tools allows researchers to sift through and analyze data effectively, providing a framework for understanding the implications of mRNA variations in sickle cell pathology and treatment strategies. Assessing the mRNA of sickle cell hemoglobin against an ever-growing pool of data not only aids in diagnosis but also fuels the potential development of future therapies that could significantly alter treatment landscapes.
Current Research Directions
Research on sickle cell hemoglobin focuses on innovative therapeutic strategies and collaborative frameworks that promise to enhance patient outcomes and deepen our understanding. The spotlight is now on targeted therapies, which aim to correct or mitigate the effects of the abnormal hemoglobin variant. These therapies hold potential to fundamentally shift the treatment landscape of sickle cell disease. Researchers are tirelessly exploring mechanisms to target the root cause of the disease at the molecular level.
The importance of this research is highlighted as scientists strive not just to treat symptoms but to offer a curative approach. Key elements include:
- Gene Editing Technologies: Approaches such as CRISPR-Cas9 are being investigated for their potential to modify the genes responsible for producing sickle cell hemoglobin.
- Pharmacological Advances: New drugs are under study to promote fetal hemoglobin production, which can alleviate the sickling of red blood cells.
This exploration contributes to a growing body of evidence that more tailored treatments may significantly improve patient care, emphasizing the necessity for ongoing research and the substantial benefits that may arise from successful strategies.
Clinical Studies on Targeted Therapies
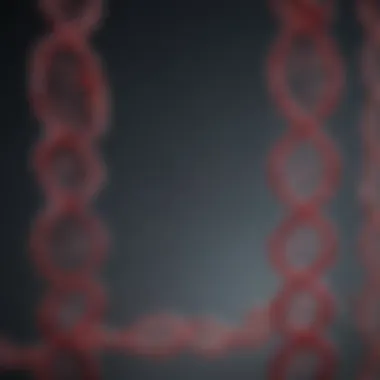
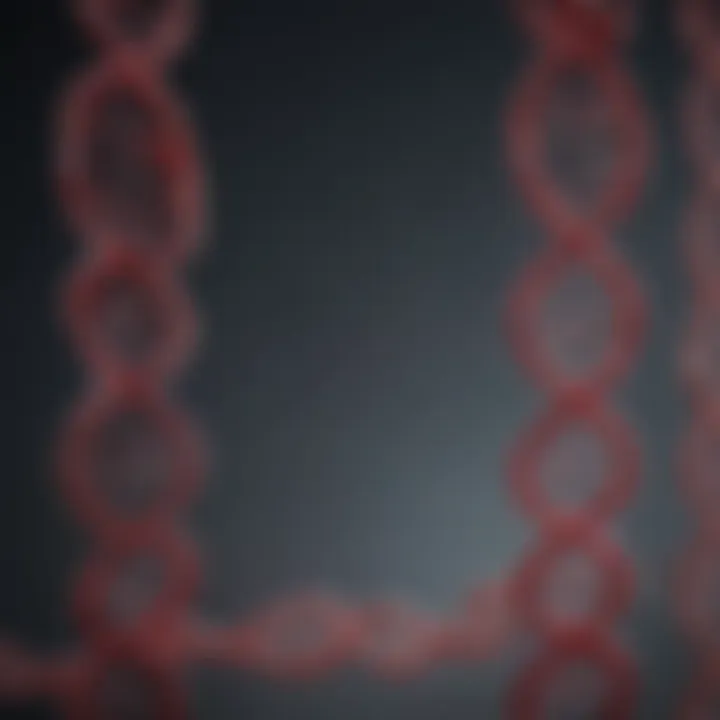
Clinical studies are at the forefront of transforming theoretical research into practical solutions. Currently, several trials are analyzing different targeted therapies aimed at sickle cell disease. These therapies are not merely supplements; they endeavor to address the underlying genetic abnormality that causes sickle cell formation. Some of the prominent approaches include:
- Gene Therapy Trials: Investigations such as the ones led by Vertex Pharmaceuticals and CRISPR Therapeutics have shown promising results in correcting the mutation responsible for sickle cell disease. Early findings suggest that patients can achieve normal levels of hemoglobin, significantly improving their quality of life.
- Fetal Hemoglobin Inducers: Compounds like hydroxyurea are researched extensively for their ability to stimulate the production of fetal hemoglobin, decreasing the sickling of red blood cells.
These clinical endeavors not only serve to test the efficacy of treatments but also foster a better understanding of patient-response variations.
Collaborative Efforts in the Global Health Network
Collaboration across the global health network has become indispensable in tackling sickle cell disease. Various organizations, academic institutions, and healthcare providers are joining forces, pooling resources and knowledge to address the multifaceted challenges presented by the disease. This unity aims to:
- Enhance Data Sharing: By consolidating data from diverse populations, the understanding of disease prevalence, treatment responses, and genetic variations may significantly expand.
- Implement Global Health Initiatives: Organizations like the World Health Organization are promoting awareness and providing frameworks for screening and management of sickle cell disease in low-resource settings.
Global partnerships not only strengthen research capabilities but also help ensure that findings translate into practical applications—making a meaningful difference in patient lives around the world.
"Research and collaboration are not merely academic exercises; they are profound commitments to enhance lives and fundamentally alter the future of health care in sickle cell disease."
In summary, the current research directions in sickle cell hemoglobin mRNA sequencing focus on targeted therapies and collaborative endeavors that carry the potential for transformative change in the treatment landscape. By propelling these efforts forward, the scientific community can further unlock vital insights that will ultimately lead to improved patient outcomes.
Comparative Insights with Other Hemoglobinopathies
Understanding sickle cell disease is not done in isolation; it opens the door to a broader conversation about various hemoglobinopathies. Hemoglobinopathies, in general, are a group of disorders that arise from abnormalities in the structure or amount of hemoglobin produced by the body. The implications of comparing these disorders, such as thalassemia and others, are significant for both genetic research and clinical applications. It allows researchers to explore genetic variations that affect hemoglobin function and stability, providing deeper insights into therapeutic strategies across different types of anemia.
This comparative approach facilitates the identification of shared pathways and mechanisms. For example, both sickle cell disease and thalassemia arise from mutations in the hemoglobin genes, but their effects on the red blood cells and the body at large differ markedly. Recognizing these differences is crucial in developing effective targeted treatments and prevention strategies.
Thalassemia and Other Variants
Thalassemia represents a range of genetic disorders characterized by reduced production of one of the globin chains that make up hemoglobin. In contrast to sickle cell disease, where the hemoglobin produced is abnormal, thalassemia leads to an insufficient amount of normal hemoglobin. This creates a different set of clinical manifestations, including varying degrees of anemia, which can be severe, moderate, or almost undetectable.
Patients with thalassemia may experience similar symptoms to those with sickle cell disease, such as fatigue and pallor, but the pathophysiological processes diverge significantly. Here are some points of comparison:
- Genetic Mutations: Thalassemia is primarily caused by mutations affecting the alpha or beta globin chain genes. In sickle cell disease, a single change in the beta globin gene causes the formation of hemoglobin S.
- Clinical Management: Treatment for thalassemia often includes blood transfusions and iron chelation therapy to manage excess iron in the body due to repeated transfusions. In contrast, sickle cell treatment may include hydroxyurea, which helps produce fetal hemoglobin to dilute the sickle hemoglobin.
Drawing these comparisons enriches our understanding of how different mutations can result in vastly different clinical symptoms and management strategies. Both conditions underscore the importance of genetic counseling and testing, highlighting the critical role of personalized medicine in addressing hemoglobin disorders.
Evolutionary Perspectives of Hemoglobin Variations
The study of hemoglobinopathies also lends itself well to evolutionary perspectives. Mutations in hemoglobin genes are not simply deviations; they can provide survival advantages or disadvantages depending on environmental factors. It has been observed that the sickle cell trait provides a protective effect against malaria, which offers a selective advantage in certain populations, particularly in Africa.
Conversely, thalassemia mutations also arise in regions where malaria is endemic, likely due to similar evolutionary pressures. These insights show how genetics is intertwined with environmental factors and can inform public health strategies in regions affected by these diseases.
In summation, examining sickle cell disease alongside other hemoglobinopathies creates a richer understanding of the genetic underpinnings of these conditions. This approach not only sheds light on the diversity of hemoglobin disorders but also emphasizes the evolutionary significance of these mutations. By understanding these intricate interactions, researchers and clinicians can better inform their approaches to treatment, genetic counseling, and preventative measures.
End and Future Prospects
This article wraps up with a delving into the significance of understanding sickle cell hemoglobin mRNA and its broader implications for healthcare and research. The journey through the molecular landscape of sickle cell disease has revealed the nuances of how a simple mutation can lead to profound consequences. The discussion laid out here is not only a reflection on the current state of knowledge but also points toward future avenues of research and therapeutic strategies.
Synthesizing Current Knowledge
In reflecting on everything we’ve discussed, it is crucial to integrate the various aspects of sickle cell hemoglobin mRNA into a coherent understanding. The science underlying this condition illustrates the delicate interplay between genetics, biochemistry, and clinical outcomes.
- Key findings include:
- The specific mutation in the hemoglobin gene results in altered mRNA sequences.
- These mRNA variations significantly affect protein folding and functionality, leading to the hallmark sickle-shaped cells.
- Identifying these mutations opens doors for targeted therapies aimed at correcting or compensating for these defects.
The advances in mRNA sequencing technology that we explored provide powerful tools for researchers. These capabilities are pivotal for identifying mutations at a molecular level and can assist in tailoring individualized treatments that address specific genetic backgrounds.
Implications for Genetic Counseling and Treatment
The implications of this understanding extend beyond pure research into practical applications in genetic counseling and treatment avenues.
- Key considerations include:
- Genetic counseling can help inform families about the risk of sickle cell disease and the importance of carrier testing.
- Treatment may not only focus on managing symptoms but also on exploring potential therapies that correct the underlying genetic issue.
- Educational programs for patients and families about the nature of sickle cell disease and available treatments can empower them in making informed health decisions.
The prospect of gene therapy and pharmacological advancements is promising, indicating a trajectory toward more effective interventions. As we continue to gather insights from ongoing research, the potential to modify the course of sickle cell disease through innovative treatments becomes increasingly feasible.
Ultimately, the integration of knowledge from biochemistry, genetics, and clinical practice heralds the future of personalized medicine for sickle cell disease, paving the way for brighter outcomes and improved quality of life.