Understanding Diffusion: Mechanisms and Real-World Impact
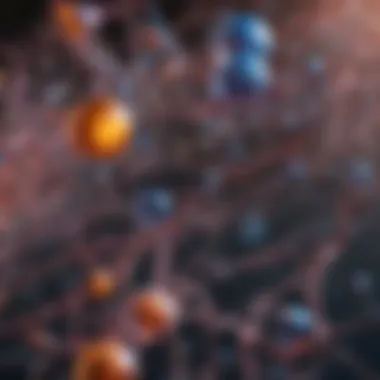
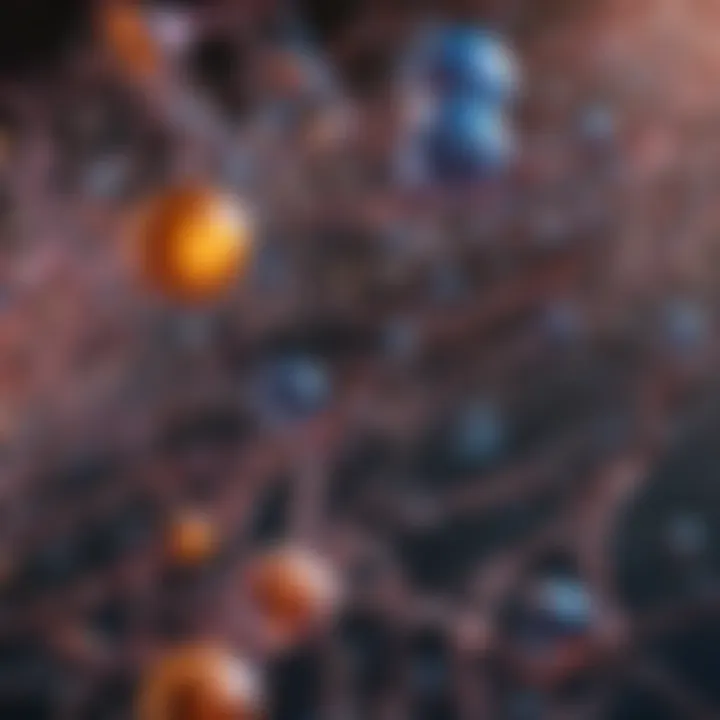
Intro
Diffusion is a process that pervades the very fabric of our universe. It’s not just a fundamental concept in science; it’s a daily reality that influences everything from the air we breathe to the technologies we develop. Often overlooked due to its seemingly straightforward nature, diffusion embodies a complexity worthy of exploration. The journey begins with understanding its fundamental principles, laying down a foundation that bridges biological systems, chemical interactions, and material science.
Understanding diffusion is like peeling an onion, revealing layers that intertwine various scientific disciplines. Each layer provides insights into why and how substances move from areas of high concentration to low concentration, driven by the natural tendency to achieve equilibrium. By dissecting these layers, we can illuminate not only the mechanisms behind diffusion but also its impact on the world.
In the following sections, we will delve deeper into the various aspects of diffusion, revealing its multifaceted role in scientific inquiry and practical applications. We shall explore:
- Key mechanisms of diffusion
- Real-world applications across different fields
- The importance of diffusion in advanced technologies
This article seeks to serve a broad audience—students, researchers, educators, and professionals—offering substantial depth of insight into the intricate processes of diffusion.
Prelims to Diffusion
In delving into the principles of diffusion, one finds that its significance stretches far broader than a mere scientific concept. Diffusion forms the basis of various natural processes and engineered systems, acting as a bridge between different mediums and states of matter. The study of diffusion gives insights not only into biological phenomena but also into chemical reactions and the behavior of materials under various conditions.
Understanding the mechanisms behind diffusion is crucial for various reasons. First and foremost, it provides the foundation for many scientific disciplines, revealing the intricate dance of particles as they move from areas of high concentration to those of lower concentration. This movement can have profound implications; for instance, in biology, it explains how cells absorb nutrients and eliminate waste. In chemistry, it guides the understanding of reaction rates and the influence of concentration variances on outcomes.
Moreover, exploring diffusion has broader societal implications. As we encounter increasingly complex challenges in technology, environmental management, and health sciences, comprehension of diffusion becomes imperative. Whether talking about pollutant dispersion in ecosystems or advancements in targeted drug delivery systems, the principles of diffusion are at play. By digging deeper into how diffusion operates, we can engineer better solutions that cater to these challenges, benefiting both our environments and ourselves.
Definition and Historical Context
Diffusion, in its simplest terms, refers to the movement of particles from a region of higher concentration to one of lower concentration, driven by random molecular motion. The term has roots that trace back to the Latin word diffundere, which means 'to spread out', perfectly encapsulating the essence of the process. Historically, understanding diffusion predates formal scientific study, with ancient philosophers postulating about the nature of mixing and blending substances.
The formalization of diffusion as a scientific principle began with the work of Robert Brown in the early 19th century through what is known as Brownian motion. This observed random movement of particles suspended in liquids ignited further interest and laid the groundwork for mathematical models. Following Brown, scientists such as Adolf Fick expanded on these ideas, articulating Fick's laws of diffusion, which quantify the rate and mechanisms involved in the process.
In modern science, diffusion is viewed through the lens of various disciplines. In biology, it is integral to understanding cellular dynamics; in physics, it sheds light on thermodynamic principles; and in materials science, it links to the properties and behaviors of various substances. The evolution of diffusion studies captivates scholars, providing a rich tapestry for future exploration and discovery.
Importance of Studying Diffusion
The importance of studying diffusion cannot be overstated. Applications can be seen deeply woven into the fabric of our daily lives. For instance, consider how diffusion plays a role in how we breathe. Oxygen enters the bloodstream through the alveoli in our lungs via diffusion, illustrating its key role in sustaining life. In a more abstract sense, by studying diffusion, researchers can manipulate conditions to enhance or control these processes, leading to innovations in medical technologies and textile engineering, among others.
Moreover, diffusion influences our understanding of environmental science. Researchers look into how pollutants diffuse in water systems to devise effective cleanup methods and mitigate ecological damage. Similarly, foaming agents in food products, ointments, and many consumer goods depend on diffusion for effective performance. This cross-disciplinary relevance highlights how the principles of diffusion touch on everyday experiences and advanced technological innovations alike.
"The study of diffusion is not merely academic; it’s an exploration of the fundamentals underlying life, existence, and progress across various fields."
In closing, the inquiry into diffusion is not simply restricted to theoretical knowledge. It comes with practical benefits and implications that extend into various aspects of life, technology, and the environment. Understanding diffusion enhances our capability to innovate and creatively tackle problems hounding our societies today.
Fundamental Principles of Diffusion
Understanding the fundamental principles of diffusion is essential for grasping its wide-ranging implications in various scientific fields. These principles not only lay the groundwork for explaining how substances move from one area to another but also drive multiple applications in biology, chemistry, and material science. By delving into these principles, researchers and professionals can navigate the complexities of diffusion phenomena and apply this knowledge effectively in different contexts.
Fick's Laws of Diffusion
Fick's Laws serve as the backbone of diffusion theory. The first law states that the rate of diffusion of a substance is directly proportional to the concentration gradient. This means that the steeper the concentration difference between two regions, the faster the substance will diffuse from high to low concentration.
The second law, on the other hand, relates to how the concentration of the substance changes over time. It describes the temporal aspect of diffusion, indicating how, over a given period, the concentration of a substance will equilibrate throughout the space. Together, these laws form a robust framework for predicting how substances behave under various conditions. For instance, in medicine, these laws can help in designing effective drug delivery systems by optimizing how quickly a drug disperses in the body.
Concentration Gradients
Concentration gradients are at the heart of diffusion processes. Imagine you inadvertently spill a bit of perfume in a room. Initially, the area around the spill is awash with fragrance, while corners of the room smell faintly, if at all. The difference in smell is a concentration gradient, which drives the scent to spread until it’s evenly distributed throughout the air.
In scientific terms, a concentration gradient exists when a substance's density varies across a space. This gradient is crucial because it dictates the direction and rate of diffusion—substances will always migrate from areas of higher concentration to those of lower concentration until they achieve a state of equilibrium. This concept plays an integral role in biological systems, such as during gas exchange in the lungs, where oxygen moves from the alveoli into the blood, following a concentration gradient.
Diffusion Coefficients
Diffusion coefficients are a key measure that determines how quickly diffusion occurs. They take into account various factors, including temperature, viscosity of the medium, and the size of the diffusing particles. A higher diffusion coefficient implies that particles spread out quickly, while a lower coefficient indicates slower diffusion.
For example, consider how food coloring disperses in water. If you drop a drop of dye in a glass of warm water, it spreads rapidly. This rapid dispersion is due to a higher diffusion coefficient in warmer water compared to cold. This coefficient is often represented in calculations related to Fick's Laws, enabling scientists to predict how substances would diffuse in different scenarios.
"Diffusion is not just a chemical phenomenon; it is the very fabric of life processes, bridging numerous disciplines and applications."
Understanding and applying these fundamental principles pave the way for advancements across various fields—from enhancing crop yields in agriculture through optimized nutrient diffusion, to engineering more effective medical treatments via targeted drug delivery systems. Each principle intertwines with practical applications, emphasizing the pervasive relevance of diffusion in the natural and engineered world.
Types of Diffusion Processes
Understanding the various types of diffusion processes is crucial, as they lay the groundwork for many natural phenomena and technological advancements. Each type serves a specific role, influencing how substances move across different mediums, which is integral not just in biology, but also in materials science, chemistry, and environmental science. By grasping these processes, students and researchers can better appreciate how diffusion shapes the world around us, from the nutrient uptake in cells to the operation of advanced manufacturing technologies.
Simple Diffusion
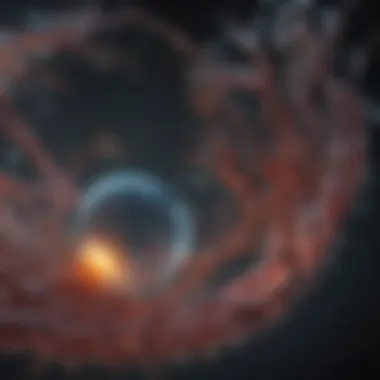
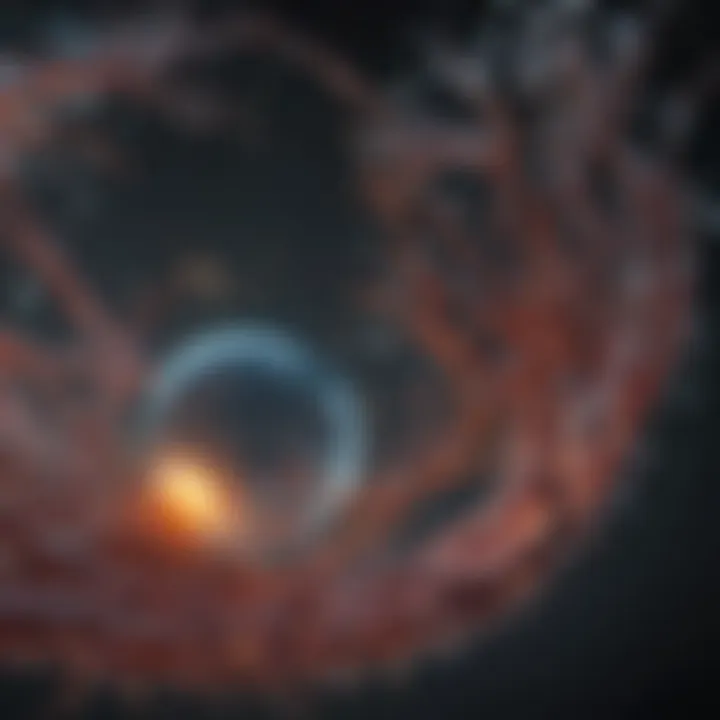
Simple diffusion is perhaps the most straightforward type of diffusion. It refers to the movement of molecules from an area of higher concentration to an area of lower concentration without the assistance of proteins or energy input. This process is driven solely by the concentration gradient and is essential for maintaining balance within biological systems.
For instance, the exchange of oxygen and carbon dioxide in the lungs primarily occurs through simple diffusion. When we inhale, the concentration of oxygen is higher in the alveoli than in the blood, prompting oxygen molecules to diffuse into the bloodstream. Conversely, carbon dioxide moves from the blood into the alveoli to be exhaled. This type of diffusion is not only limited to gases; it also applies to small, nonpolar molecules, such as lipids passing through cell membranes.
Facilitated Diffusion
Facilitated diffusion takes things up a notch by utilizing specific protein channels in membranes to assist the transport of substances. Unlike simple diffusion, it involves larger, polar, or charged molecules that can’t simply pass through the lipid bilayer. These proteins act as gateways, allowing molecules like glucose or ions to enter and exit cells effectively.
For example, the transportation of glucose into cells is a classic case of facilitated diffusion. Glucose can’t diffuse through the cell membrane on its own, so it relies on a specific transporter protein. As the concentration of glucose is higher outside of the cell, the protein facilitates the movement into the cell, allowing it to be utilized for energy production. This method is crucial in maintaining cellular function and energy balance, especially after eating.
"Facilitated diffusion is like a toll booth easing traffic flow—allowing only certain vehicles through on a busy highway."
Active Transport
In stark contrast, active transport requires energy, usually in the form of ATP, to move molecules against their concentration gradient—from areas of lower concentration to areas of higher concentration. This process is vital for maintaining essential concentrations of ions and nutrients inside cells, which is important for functions like nerve impulse transmission and muscle contraction.
A well-known example of active transport is the sodium-potassium pump. This mechanism pumps sodium ions out of the cell and potassium ions into the cell against their respective concentration gradients. By doing so, it establishes a gradient that is critical for a variety of cellular functions, including maintaining osmotic balance and facilitating electrical signaling in nerve cells.
Osmosis
Osmosis is a special case of diffusion that specifically involves water molecules. Water moves through semipermeable membranes from an area of lower solute concentration to an area of higher solute concentration, aiming to equalize concentrations on both sides. This process is incredibly important for maintaining cell turgor in plants and the overall balance of fluids in our bodies.
For instance, in a plant cell, if the external environment has a higher concentration of solutes (hypertonic solution), water will exit the cell, leading to wilting. Conversely, if placed in a lower solute concentration solution (hypotonic), water enters the cell, causing it to swell and potentially burst. Understanding osmosis is fundamental in fields like agriculture and medicine, where the water balance of cells can dictate health and productivity.
In summary, these types of diffusion processes highlight the complexity and importance of molecular movement across barriers, laying the foundation for many biological, chemical, and environmental interactions. Each process carries unique characteristics and implications, which can contribute significantly to our understanding of life and technology.
Molecular Dynamics of Diffusion
Molecular dynamics play a crucial role in understanding diffusion by elucidating how molecules move and interact at microscopic levels. This area of study not only deepens our comprehension of diffusion phenomena but also lays a foundation for practical applications across various scientific fields. When discussing diffusion, one cannot overlook its molecular intricacies, where temperature and pressure can dramatically influence the behaviors of substances.
Role of Temperature and Pressure
Temperature and pressure significantly impact molecular motion. In a nutshell, as temperature increases, the kinetic energy of molecules escalates. Think of it like a crowded room, where people start jostling each other more when the heat is turned up. Higher molecular velocities lead to enhanced diffusion rates, allowing substances to spread more rapidly.
Conversely, changes in pressure can compress or expand materials, affecting how closely molecules are packed together. Increased pressure often reduces the space between molecules, which can consequently inhibit diffusion. Whether in biology or material science, understanding these dynamics is vital in optimizing processes such as drug delivery or enhancing material properties.
"Temperature and pressure are like the sun and wind; they shape the landscape of molecular motion and diffusion patterns."
Interactions at the Molecular Level
At the heart of diffusion lies the interaction at the molecular level, where every atom and molecule communicates in its own silent language. The type of interactions largely determines how substances diffuse. For instance, in simple diffusion, molecules move autonomously from areas of high concentration to low concentration, kind of like water flowing downhill. However, in cases involving facilitated diffusion, carrier proteins or channels assist in transporting substances across membranes, applicable for larger or charged molecules.
The nature of bonding, whether ionic, covalent, or hydrogen, intricately governs the speed and efficiency of diffusion. This interplay can affect how quickly nutrients are absorbed in cells or how environmental pollutants spread in ecosystems. It's not just about the molecules themselves but also how they react and interact with their surroundings.
- Key Factors Influencing Interactions:
- Molecular size and mass
- Type of bonding present
- Environmental conditions (temperature, pressure)
Understanding these interactions is essential for advancing technologies in areas such as drug formulation and environmental remediation. By honing in on the microscopic behaviors of molecules, researchers can design more effective systems and processes.
So, the intricacies of molecular dynamics within diffusion aren't just details; they're the very framework of understanding how materials interact in both nature and technology.
Applications of Diffusion in Biology
The application of diffusion in biology serves as a cornerstone for various fundamental biological processes. Understanding diffusion's mechanisms not only sheds light on the behavior of molecules within living organisms but also illustrates how life sustains itself through intricate interactions. This section explores several vital applications of diffusion, emphasizing its role in cellular functions, nutrient dynamics, and gas exchange.
Cellular Processes
Diffusion is a critical player in cellular processes, primarily because it facilitates the movement of substances across cell membranes. Cells engage in diffusion to maintain homeostasis, where the concentration of ions and molecules inside the cell must stay relatively stable compared to the external environment.
- Selectivity: Cell membranes are selectively permeable, allowing only certain molecules to pass through. For example, small, nonpolar molecules such as oxygen and carbon dioxide diffuse freely due to their size and solubility.
- Significance of Concentration Gradients: The concentration gradient drives the diffusion process. When there's a higher concentration of a substance outside the cell than inside, the substance will move into the cell until equilibrium is achieved. This principle is vital for many biological reactions, including the transport of essential nutrients and ions required for cellular function.
This process is not just passive; rather, it's integral to how cells operate. The movement of water, ions, and vital nutrients through diffusion supports energetic processes like ATP production in mitochondria and cellular respiration.
Nutrient Uptake and Waste Removal
In the context of nutrient uptake and waste removal, diffusion plays a pivotal role in how organisms manage resources efficiently. Cells absorb nutrients via diffusion from their surroundings, and they expel waste products using similar mechanisms.
- Nutrient Transport: For instance, glucose from the bloodstream enters cells through diffusion. After ingestion, food is broken down into simpler molecules, and those smaller molecules diffuse into cells. This process not only emphasizes efficiency but also illustrates the simplicity of nutrient uptake.
- Waste Excretion: Conversely, cells remove waste products—such as carbon dioxide and urea—back into the surrounding environment using diffusion. The concentration gradient of waste products is crucial here; as concentrations drop inside the cell, molecules move outwards to rectify the imbalance.
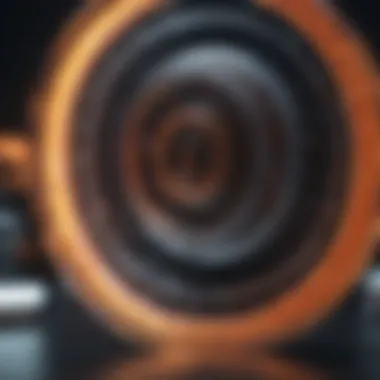
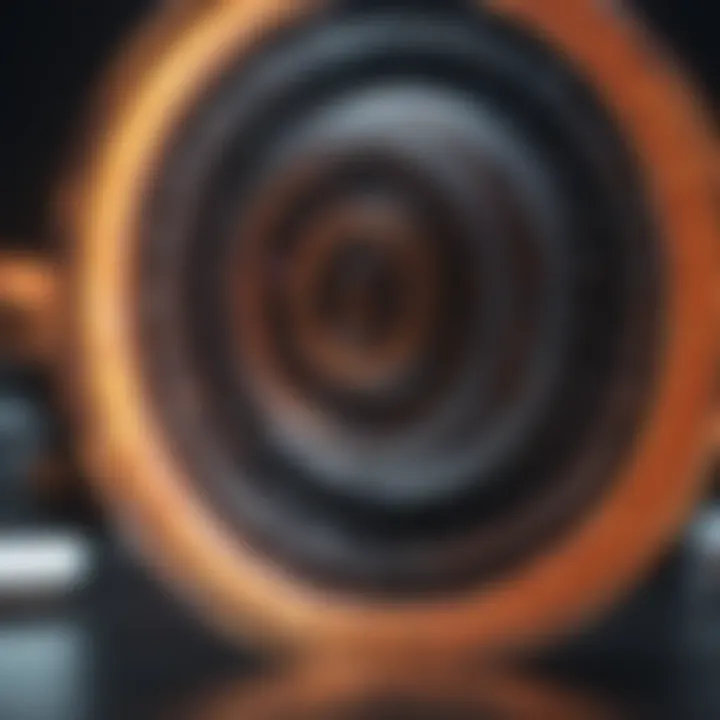
Both processes are crucial for maintaining not only cellular health but also the overall functionality of complex multicellular organisms. Effective nutrient uptake and waste removal directly impact overall growth, reproduction, and the maintenance of homeostatic conditions.
Gas Exchange in Respiration
Gas exchange, essential for respiration, is another vital application of diffusion in biology. In higher organisms, diffusion enables the transfer of gases—specifically, oxygen and carbon dioxide—between the atmosphere and the cells.
- Alveolar Diffusion: In humans, for instance, oxygen molecules diffuse from the alveoli in the lungs into the bloodstream, while carbon dioxide diffuses in the opposite direction to be exhaled. The thin walls of the alveoli and surrounding capillaries create an ideal environment for efficient gas exchange. The surface area available for diffusion is maximized, promoting swift gas transfer.
- Efficiency: This mechanism allows organisms to respire efficiently, as the need for oxygen and the removal of carbon dioxide are critical for energy metabolism. The effectiveness of gas exchange underlines the importance of diffusion in sustaining life.
In summary, the intricate roles of diffusion in various biological processes—from cellular functions to the management of nutrients and gas exchange—highlight its significance in maintaining life. Understanding these applications underpins our knowledge of biological systems, enhancing our comprehension of health, disease, and biotechnological advances.
"Diffusion isn't just an essential process; it's the very pulse of life at the molecular level, driving not just cells but whole organisms."
By grasping the complex interplay of diffusion within biology, one can appreciate the brilliance of life’s design—efficient, responsive, and adaptable.
Diffusion in Chemistry
When it comes to the realm of chemistry, diffusion plays a pivotal role that often goes unnoticed by those who aren’t knee-deep in scientific studies. This intricate process isn't just about mixing substances; it's a fundamental mechanism that underpins various phenomena within chemical reactions and the behavior of materials.
Understanding diffusion in chemistry facilitates insight into reaction dynamics, impacting everything from industrial processes to biological functions. It serves as a vital bridge between theoretical concepts and practical applications in laboratories and industries alike.
Kinetics of Chemical Reactions
The kinetics of chemical reactions refer to the rates at which reactants convert into products. Diffusion emerges as a key player here. In many reactions, particularly those in solutions, how quickly reactants can move towards each other dictates how fast the reaction occurs. The concentration of reactants and their ability to mingle effectively can significantly alter reaction speeds.
- Diffusion Rate: A critical factor affecting reaction time is the diffusion rate. The greater the concentration gradient, the higher the rate at which molecules will scatter, thereby accelerating the reaction.
- Influence of Temperature: Increasing temperature can enhance molecular movement, subsequently boosting the diffusion rate—a critical consideration in reaction kinetics.
“In the world of chemistry, a minute shift in diffusion can lead to monumental changes in the outcome of reactions.”
In instances where reactants are gases, diffusion becomes even more pronounced. For instance, during combustion reactions, the rate of diffusion can dictate not only the energy efficiency of the reaction but also the nature of the products formed.
Role in Catalysis
Catalysis, the process that increases the rate of a chemical reaction via a substance that is not consumed during the reaction, relies heavily on diffusion. This role is vital, particularly in heterogeneous catalysis, where reactants can encounter catalysts that are in a different phase (like gas or liquid interacting with a solid).
- Surface Diffusion: Molecules must diffuse across the surface of the catalyst to react effectively. The efficiency of this movement can affect the overall efficiency of the catalyst.
- Intrusion into Catalyst Pores: In many catalytic processes, reacting molecules need to penetrate the porous structure of a catalyst. The rate of this intrusion is directly linked to diffusion dynamics.
In such contexts, diffusion is not merely an abstract concept but a tangible factor influencing the performance of catalysts in industrial settings. Enhanced diffusion can lead to faster reactions, which can significantly lower production costs and maximize yield, ushering in more sustainable practices in chemical manufacturing.
Diffusion in Material Science
Diffusion in material science plays a crucial role, serving as the backbone for understanding how materials behave and interact on a microscopic scale. It’s this movement of particles that determines a material's properties, influencing everything from strength to thermal conductivity. Material scientists study this phenomenon to devise stronger, lighter, and more efficient materials, essential for advancements in technology and industry.
Think of diffusion like the subtle currents beneath the surface of a still pond. It’s not always visible, but it shapes what we see above.
Transport Properties of Materials
Transport properties refer to how different materials allow particles, heat, and electricity to pass through them. This includes understanding how quickly ions, atoms, or molecules can move within a material. Several factors come into play in these transport properties:
- Porosity: This is the measure of void spaces in a material. High porosity often leads to increased diffusion rates, allowing substances to move through more freely.
- Temperature: Generally speaking, as temperature rises, particle movement increases, leading to enhanced diffusion. Hotter materials tend to be more permissive to diffusion processes.
- Material Structure: The arrangement of atoms or molecules within a material can vastly affect how efficiently diffusion occurs. For instance, a crystalline structure may hinder movement compared to an amorphous structure due to tightly packed atoms.
This interplay of factors not only helps in theoretical calculations but also has vast implications in practical applications like alloy manufacturing and polymer development.
Applications in Semiconductor Manufacturing
In the realm of semiconductor manufacturing, diffusion takes on an even more significant role. It’s vital for shaping electronic devices, with semiconductors being the lifeblood of modern electronics. Here are some notable aspects of diffusion in this field:
- Doping: Diffusion is used to introduce impurities into semiconductors. This process, called doping, adjusts the electrical properties of the materials, making them conductive, insulative, or semi-conductive, depending on needs.
- Thin Film Deposition: During the fabrication of thin films, which are essential in many devices, controlled diffusion ensures uniform coatings and functionality. The diffusion process impacts how materials adhere and their resulting characteristics.
- Defect Control: Understanding how atoms diffuse helps in managing defects within semiconductors. These defects can significantly impact device performance. Effective diffusion management can minimize defects, leading to higher yield rates and functional devices.
"Diffusion in semiconductor manufacturing isn't just a phenomenon; it's the orchestrating principle that dictates the harmony of modern electronic systems."
As researchers continue to probe deeper into the nuances of diffusion, the promise of improved materials and technologies remains a dominant focus. The interplay between theoretical knowledge and practical application can drive further innovations, paving the way for the future of the semiconductor industry.
Environmental Impact of Diffusion
The environmental impact of diffusion is both substantial and multifaceted. Understanding how substances move through different media is crucial for addressing ecological challenges and managing resources. It not only affects the livelihood of countless organisms but also has implications for human health and climate stability. The processes of diffusion can be seen as threads weaving through ecosystems, connecting chemical interactions, biological responses, and physical changes in real-time.
- Key Aspects to Consider:
- The movement of pollutants through air, water, and soil.
- Ecosystem responses to these pollutants at the molecular level.
- The role diffusion plays in mitigating or exacerbating environmental issues such as climate change.
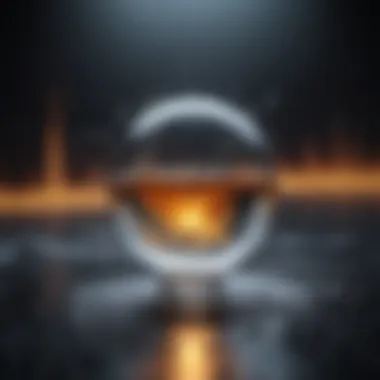
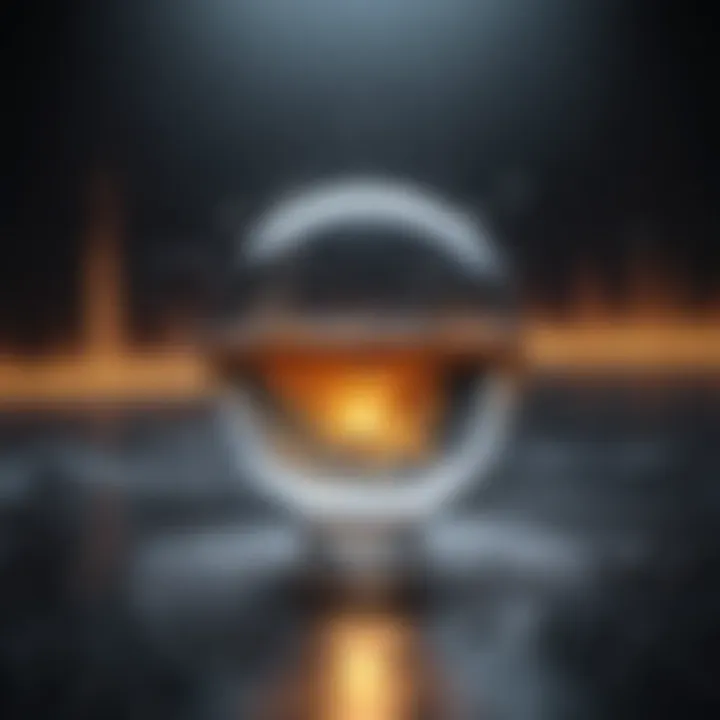
Examining the environmental impact of diffusion can lead to better policies and practices aimed at protecting both biodiversity and human interests.
Pollutant Transport in Ecosystems
Pollutant transport via diffusion is a vital matter, as it dictates how contaminants spread through different environments. In aquatic systems, for example, a drop in a river can radiate outward, leading to a cascade of biological reactions. Chemical substances, especially heavy metals and other toxic compounds, diffuse from high concentration areas to lower ones, influencing organisms that might be miles away from the original source.
The environmental regulations often consider diffusion rates when assessing how quickly pollutants can disperse. This knowledge informs cleanup responses and helps in predicting the potential risks to wildlife and human populations. For instance:
- Key Considerations:
- Bioaccumulation: Many toxic agents can accumulate in the food chain. Fish consumed by humans might absorb more pollutants over time, affecting health.
- Long-Range Transport: Pollutants transported over long distances can cause widespread damage. An example is how atmospheric pollutants can travel across states or even countries.
- Mitigation Strategies: Understanding diffusion can guide the development of barriers or remediation techniques to contain pollutant spread.
"Understanding how pollutants diffuse is like reading a complex story—each chapter builds on the previous, illustrating a connection that can impact the future of ecosystems."
Role in Climate Change
Diffusion plays an often unseen, yet essential role in climate change. Gases like carbon dioxide and methane enter the atmosphere through various processes, and their diffusion shapes the rates of global warming. Their concentrations in different pockets fluctuate as they disperse, creating imbalances that drive weather patterns.
Furthermore, the ocean acts as a tapestry of diffusion. It absorbs excess CO2, which affects marine life and further complicates climate dynamics. Here are a few critical facets:
- Key Impacts:
- Gas Exchange: The diffusion of gases between the atmosphere and the ocean engages in a delicate balance that influences climate stability.
- Ocean Acidification: Increased absorption of CO2 leads to chemical changes in ocean water, affecting marine organisms' ability to survive.
- Feedback Loops: Changes in diffusion rates may create loops where increased temperatures result in greater emissions of greenhouse gases, further driving climate change.
Understanding the diffusion processes helps researchers and policymakers project future scenarios and devise effective environmental strategies. Education on diffusion can foster solutions to counteract environmental deterioration, ensuring a more sustainable future.
Challenges in Modeling Diffusion
Modeling diffusion is no walk in the park. It's a complex endeavor that requires a thorough understanding of both fundamental principles and practical applications. The importance of discussing the challenges in modeling diffusion lies in the fact that our ability to accurately predict and analyze diffusion processes can have lasting impacts across various scientific and engineering fields. From accurately simulating pollutant dispersion in the environment to designing effective drug delivery systems, overcoming the challenges in diffusion modeling can greatly enhance our effectiveness in tackling real-world problems.
This section outlines significant considerations that highlight the difficulties faced in diffusion modeling:
- Complexity of Systems: Biological, chemical, and physical systems that exhibit diffusion are often multi-faceted and heterogeneous. For instance, the flow of nutrients within a soil matrix is not a simple event. Various factors, including soil composition and moisture content, influence how effectively substances diffuse. Understanding these systems means accounting for a multitude of variables that can complicate the modeling process.
- Temporal and Spatial Variability: Diffusion does not happen in a vacuum. Environmental conditions, such as temperature fluctuations or changes in pressure, contribute to temporal and spatial variability in diffusion processes. Modeling these varying conditions can create additional layers of difficulty, often necessitating adaptive models that can respond to real-time data.
- Scale Issues: The scale of interest is crucial when modeling diffusion. What works in a microscale setting may not necessarily translate to a macroscale perspective – think of how nutrients diffuse in a single cell versus an entire organism. This disparity can lead to misinterpretations if scaling isn't considered carefully.
The pitfalls that emerge from these complexities can mislead researchers. Thus, acknowledging these modeling challenges is essential for improving the accuracy of predictions and enhancing the utility of diffusion models in practical applications.
Mathematical Formulations
Mathematical formulations are the backbone of diffusion modeling, serving as a structured method to quantify and simulate the behavior of diffusing substances. Commonly used models include:
- Fick's Laws: These laws provide a quantitative description of diffusion, establishing fundamental relations among concentration gradients, flux, and diffusion coefficients. Despite their efficacy, they often require simplifications that can obscure the reality of complex systems.
- Partial Differential Equations: These equations describe how the concentration of a substance changes over time and space. While powerful, they can become unwieldy, particularly when non-linear behaviors or additional forces come into play.
- Stochastic Models: These frameworks account for random variations within diffusion processes. They can become essential when dealing with systems where uncertainty is a default state, such as differences in individual molecular movements.
Addressing the intricacies of each mathematical formulation involves expertise and often trial-and-error. For many researchers, the road can be long and winding.
Computational Simulations
Simulations play a pivotal role in diffusion modeling as they enable the exploration of scenarios that might be challenging—or even impossible—to study experimentally. Employing computational tools facilitates a deeper understanding of diffusion phenomena through various avenues:
- Multiscale Simulations: These allow researchers to tackle diffusion processes at multiple scales simultaneously, incorporating information from molecular dynamics to continuum models. This multi-layered perspective can offer insights that a single-scale analysis might miss.
- Monte Carlo Methods: This stochastic approach uses random sampling to solve problems that might be deterministic in principle. By simulating numerous possible outcomes, Monte Carlo methods provide a robust perspective on diffusion behavior.
Utilizing computational simulations can yield significant advantages, but they also come with challenges such as:
- Computational Costs: Running detailed simulations often requires substantial processing power and time. This need can limit the scope and scale of analyses that researchers can feasibly undertake.
- Model Validation: Simulations must be compared against experimental data to ensure their reliability, but generating accurate data can also pose its own obstacles.
In summary, tackling the challenges in modeling diffusion, both mathematically and computationally, will enhance our understanding of this fundamental process. As we continue to refine our approaches, the insights gained can lead to significant advancements across numerous disciplines.
Future Directions in Diffusion Research
Exploring the future of diffusion research is vital not just for scientists but also for industries and society at large. The implications of ongoing research in this area can reshape how we understand processes that range from cellular functions to innovative materials. As we gear up for the next decade, several emerging trends and technologies are poised to redefine our approaches and applications of diffusion.
Advances in Nanotechnology
Nanotechnology stands as a forefront player in the evolving landscape of diffusion. As materials shrink to the nanoscale, the rules that govern diffusion do not merely scale down; they transform. For instance, the behavior of particles less than 100 nanometers can differ significantly due to quantum effects, surface area-to-volume ratios, and changes in thermodynamic properties. These factors lead to new diffusion pathways and mechanisms that challenge traditional models.
- Enhanced Transport: One of the most promising aspects of nanotechnology is its role in enhancing the transport properties of materials. Nanomaterials, such as carbon nanotubes and graphene, exhibit remarkably efficient diffusion rates, thus being useful in various applications from electronics to energy storage.
- Targeted Delivery: In fields such as drug delivery, nanoparticles are engineered to navigate biological environments with precision. These particles can facilitate controlled release systems that allow medications to diffuse only when they reach specific cells or tissues, dramatically improving therapeutic outcomes.
- Diverse Applications: Moreover, the advances in nanotechnology find applications in environmental remediation, where nanoscale materials can help absorb contaminants more effectively due to their increased surface area.
Implications for Drug Delivery Systems
In the realm of pharmaceutical sciences, understanding diffusion is crucial, especially in the development of advanced drug delivery systems. These systems harness the principles of diffusion to optimize how and when medications are administered.
- Controlled Release Mechanisms: Modern drug delivery systems are increasingly sophisticated. Functional polymers can respond to environmental stimuli—like pH or temperature—facilitating a controlled diffusion of drugs at just the right time and place. This can substantially reduce side effects and enhance the drug’s efficacy.
- Personalized Medicine: As personalized medicine gains traction, the ability to tailor drug delivery systems to individual patients hinges on a deep understanding of diffusion dynamics. Researchers are looking at how genetic profiles and metabolic rates affect drug distribution and uptake in the body.
"The advances in drug delivery systems not only illustrate the nuances of diffusion but also promise to revolutionize treatment paradigms across numerous medical fields."
- Cross-Disciplinary Collaboration: The integration of disciplines—biotechnology, materials science, and computational modeling—brings new perspectives to diffusion research. Collaborative efforts can catalyze innovations, from designing nanoparticles that can diffuse through cell membranes to creating hydrogels that release drugs based on patient-specific requirements.
In summary, as we draw closer to the future, diffusion research is set to unlock remarkable potentials across various fields. Nanotechnology and drug delivery systems are not just buzzwords; they represent a paradigm shift in how we address some of society’s most pressing needs, bridging chemical insight with practical application.