Understanding Lithium Ion Battery Mechanisms
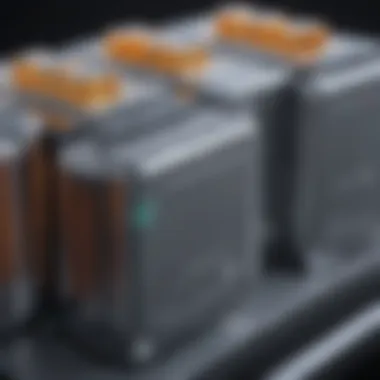
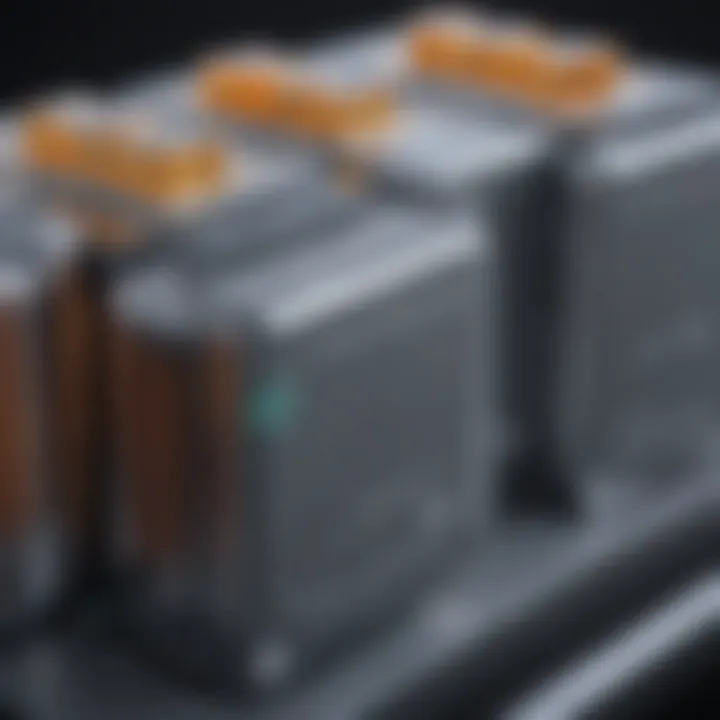
Intro
Lithium ion batteries have become a cornerstone of modern portable technology. Their significance extends beyond personal gadgets; they power electric vehicles and support renewable energy systems. This article intends to delve into the fundamental mechanisms that drive lithium ion batteries. Understanding these mechanisms is essential not just for researchers, but also for engineers and students pressed to innovate in energy storage solutions.
By examining the composition, charge and discharge cycles, and how these processes influence efficiency and longevity, we pave the way for a comprehensive analysis. We also touch upon recent advancements and what the future holds for this crucial technology. Through a detailed exploration, we aim to equip the reader with a robust framework for understanding lithium ion batteries and their broader application in contemporary society.
Research Overview
Summary of Key Findings
In exploring lithium ion batteries, several key points arise:
- Ion Movement: The movement of lithium ions between the anode and cathode is critical for the battery's functionality during both charging and discharging phases.
- Material Composition: The choice of materials for the electrodes and electrolyte fundamentally impacts battery performance, efficiency, and life span.
- Efficiency Factors: Parameters such as temperature, cycle management, and material degradation play significant roles in determining the overall efficiency of these batteries.
- Technological Advancements: Innovations in materials science and battery design continue to enhance the capabilities and applications of lithium ion batteries, including faster charging times and greater energy densities.
Background and Context
The development of lithium ion batteries began in the 1970s and received commercial viability in the 1990s. This technology emerged as a solution to the limitations of earlier battery systems, such as nickel-cadmium, which were heavier and prone to memory effects. Lithium ion batteries offered a lighter alternative with a higher energy density, which quickly made them the preferred choice in consumer electronics. Furthermore, the transition to electric vehicles created a pressing need for robust energy storage solutions, positioning lithium ion batteries as central to the future of sustainable transport.
Understanding the mechanisms behind lithium ion batteries not only serves to advance research but also informs practical applications, potentially leading to breakthroughs in electric mobility and energy storage systems.
"Lithium ion batteries are not just about powering gadgets; they are a critical component in the quest for sustainable energy solutions."
Methodology
Experimental Design
To study the mechanisms of lithium ion batteries, researchers employ multiple methodologies. These approaches often include experimental setups that mimic real-life battery usage conditions. By observing and measuring the behavior of lithium ions during charge and discharge cycles, various parameters are assessed, such as voltage, capacity, and temperature variations.
Data Collection Techniques
Data collection in battery research typically involves:
- Electrochemical Testing: Assessing the battery's voltage and current over time under controlled conditions.
- Material Analysis: Utilizing techniques like scanning electron microscopy (SEM) and X-ray diffraction (XRD) to analyze the structure and composition of battery materials.
- Cycle Testing: Evaluating how the battery performs over numerous charge and discharge cycles to understand degradation mechanisms.
These techniques not only aid in identifying existing challenges but also facilitate the development of improved lithium ion battery designs.
Prelims to Lithium Ion Batteries
Lithium ion batteries represent a significant technological advancement in energy storage systems. Their unique structure and function allow for a higher energy density compared to traditional batteries. This leads to smaller sizes and lighter weights, making them suitable for numerous applications. The benefits of lithium ion batteries include longer cycle life, lower self-discharge rates, and improved efficiency. They are critical for portable electronics, electric vehicles, and renewable energy storage solutions.
Historical Context
The development of lithium ion batteries dates back to the 1970s. Initially explored for their potential to provide higher energy outputs, the first commercial cell appeared in the early 1990s. At that time, Sony commercialized the technology, marking a pivotal moment in the field of battery chemistry. This type of battery capitalized on lithium’s light weight and high electrochemical potential. Over the years, researchers improved upon the technology, addressing issues like safety and longevity.
Significance in Modern Technology
Today, lithium ion batteries play a crucial role across various sectors. Their use in consumer electronics such as smartphones and laptops is ubiquitous. The automotive industry has also embraced these batteries; electric vehicles rely on them for efficient, long-range travel. In renewable energy, storage solutions using lithium ion batteries help stabilize fluctuations in solar and wind energy. Furthermore, as global energy demands grow, advancements in lithium ion technology may lead to more sustainable practices in energy consumption and production.
"The rise of lithium ion batteries is intertwined with modernity itself, enabling advancements that propel us into a future driven by clean energy solutions."
Basic Components of Lithium Ion Batteries
The essential elements of lithium ion batteries play a determining role in their overall performance and efficiency. Understanding the different components is crucial to grasp how these batteries function and can be improved. By scrutinizing the basic components, we can enhance our awareness of not just current technologies but also future innovations in this domain.
Anode Materials
Graphite
Graphite is the most common anode material used in lithium ion batteries. Its layered structure allows lithium ions to intercalate easily during charge and discharge cycles, a process critical for efficient energy storage. The high electrical conductivity of graphite also ensures effective electron flow. This characteristic makes it a standard choice for today's batteries.
One unique feature of graphite is its ability to maintain structural integrity after numerous cycles, which is vital for longevity in practical applications. However, it has limitations, including relatively low capacity compared to some newer materials. As battery demands increase, researchers explore alternatives that can supplement or replace graphite in the future.
Silicon Compounds
Silicon compounds present a promising alternative to graphite in anodes. The key characteristic of silicon is its remarkably high theoretical capacity, allowing for greater energy density. The potential for silicon to significantly boost capacity makes it an appealing option, especially for high-demand devices. However, silicon also presents challenges. It expands significantly during lithium intercalation, which can lead to mechanical stresses and ultimately affect the battery's performance. Addressing these challenges is a topic of ongoing research, as figuring out how to harness silicon's advantages while mitigating its disadvantages can result in more efficient lithium ion batteries.
Cathode Materials
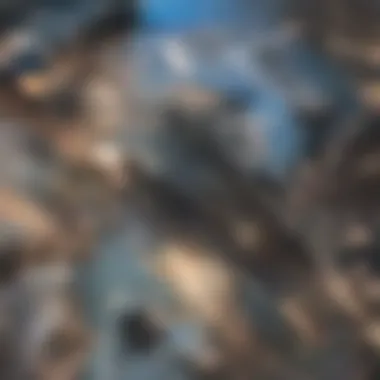
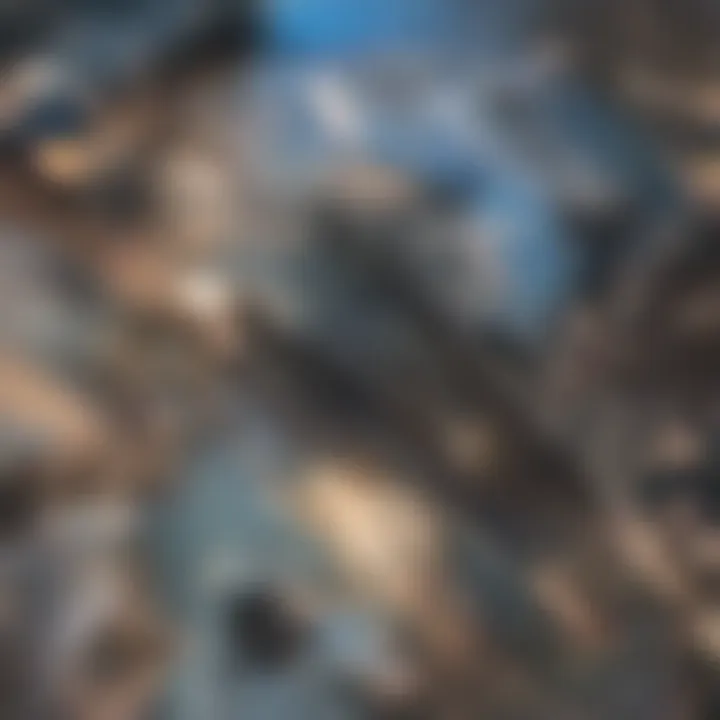
Lithium Cobalt Oxide
Lithium Cobalt Oxide is a commonly used cathode material in lithium ion batteries, particularly in consumer electronic devices. Its key characteristic lies in its good thermal stability and high energy density. This combination allows batteries to provide sufficient power while maintaining a relatively small size. The unique feature of lithium cobalt oxide is its ability to deliver high voltage, which benefits applications that require a quick energy burst, like smartphones.
Despite these advantages, lithium cobalt oxide has drawbacks, such as higher costs and environmental concerns associated with cobalt extraction. Researchers are actively seeking alternatives that can provide similar benefits without the negative implications.
Lithium Iron Phosphate
Lithium Iron Phosphate is another notable cathode material, especially in electric vehicles and renewable energy systems. One of its significant advantages is its thermal stability and safety, minimizing risks of overheating or fire. This makes lithium iron phosphate favored for applications where safety is paramount.
A unique feature is its lower energy density compared to lithium cobalt oxide. However, its other advantages may make it more viable in specific applications. Its durability and longevity are often cited as major benefits, extending the lifecycle of the batteries that use it. As the demand for safe and reliable energy storage increases, lithium iron phosphate rides a wave of interest.
Electrolyte Composition
The electrolyte is another vital element in lithium ion batteries. It is responsible for facilitating the movement of ions between the anode and cathode. Typically, a lithium salt dissolved in an organic solvent creates an electrolyte that simultaneously provides ionic conductivity while maintaining electrochemical stability. Choosing the right electrolyte composition can significantly affect battery performance, safety, and lifespan, underscoring its importance in the overall functionality. Current research focuses on optimizing these compositions to improve performance and reduce flammability.
Separator Functionality
Separators play a crucial role in preventing direct contact between the anode and cathode, which could lead to short-circuits. Usually made from microporous materials, separators allow lithium ions to pass through while blocking electron flow. The quality of the separator influences battery efficiency and safety. A well-designed separator can enhance battery life while reducing potential hazards. As lithium ion technology advances, the exploration of new materials and designs continues in an effort to improve separator effectiveness and reliability.
Principles of Operation
The principles of operation for lithium ion batteries are essential in expanding our understanding of these energy storage systems. This section looks into how batteries operate during both charge and discharge cycles, focusing on the crucial movements of ions and electrons. By comprehending these principles, one can better appreciate efficiency, performance, and the potential challenges that may arise.
Charge Process
Ion Movement
Ion movement in lithium ion batteries is a core aspect that influences overall efficiency. During charging, lithium ions move from the cathode to the anode through the electrolyte. This process is vital as it allows for energy storage within the battery. The key characteristic of ion movement is its speed, which can impact the rate of charging. Fast ion movement is beneficial as it reduces the time needed to fully charge the battery, which is a desirable quality in modern devices.
One unique feature of ion movement is its dependence on temperature. At higher temperatures, ion mobility increases which can enhance charging speed. However, this can also lead to potential risks, such as thermal runaway.
Advantages of effective ion movement include increased energy density and improved overall battery performance. However, the trade-off is the potential for reduced lifespan if the battery is subjected to extreme conditions.
Electron Flow
Electron flow is equally significant in the charging process. While lithium ions travel towards the anode, electrons move through the external circuit from the cathode to the anode. This flow generates electric current, thus ensuring that the device operates effectively. The crucial characteristic of electron flow is that it is quick and allows for immediate power delivery to devices being charged.
A distinct feature of electron flow is its linear path in the external circuit, which facilitates a steady supply of current. It allows electronic devices to function optimally during the charging process. Yet, the increased demand for higher electron flow can sometimes result in overheating, which may affect battery longevity.
Discharge Process
Energy Release Mechanism
The energy release mechanism happens when the battery discharges its stored energy. Lithium ions move back from the anode to the cathode, and in this process, electrons flow through the external circuit to deliver energy to connected devices. This mechanism is crucial for the functionality of lithium-ion batteries, serving as the bridge connecting stored energy and practical application.
A key characteristic here is the efficiency of the energy release. High energy discharge efficiency translates to more usable energy from the battery, thus improving the performance of devices. A unique feature of this mechanism is the voltage maintained during discharge, which can fluctuate based on temperature and load demand. Effective management of this can prevent energy wastage.
The advantages of a reliable energy release mechanism include long-duration power supply and high performance during discharge periods. Nonetheless, improper management might lead to reduced cycle life and potential damage to the battery.
Voltage Behavior
Voltage behavior during discharge provides insight into the performance of a lithium ion battery. The voltage determines how much energy can be extracted from the battery at any given time. Typically, a stable voltage is desirable, as it reflects consistent energy delivery. A notable characteristic of voltage behavior is its relationship with the state of charge. As the battery discharges, voltage decreases, which can signal to users an approaching need for recharging.
One unique aspect of voltage behavior is the existence of voltage sag under high load. This effect can limit performance, particularly in power-intensive applications. Strategies like choosing proper load settings can help mitigate such issues, ensuring that voltage levels remain stable throughout discharge cycles.
In summary, understanding the principles of operation—particularly focusing on ion movement, electron flow, and related mechanisms—offers a clear view of how lithium ion batteries function. Insights into these processes highlight their significance in device performance and the challenges faced in optimizing battery life and efficiency.
Thermodynamics of Lithium Ion Batteries
The thermodynamics of lithium ion batteries play a crucial role in understanding their operational efficiency and overall performance. This section explores the thermal dynamics involved in various processes, including heat generation during operation and the impacts on battery lifespan. Understanding these thermodynamic principles helps in designing better batteries and optimizing their use in a wide range of applications.
Heat Generation During Operation
One of the significant aspects of lithium ion batteries is the heat generation that occurs during both charging and discharging processes. This heat is primarily a result of internal resistance and electrochemical reactions within the battery. When lithium ions move between the anode and cathode, energy is dissipated as heat. This can be attributed to several factors:
- Internal Resistance: The flow of ions through the electrolyte and conductive materials leads to energy loss, which manifests as heat.
- Electrochemical Reactions: The reactions involved in lithium intercalation and deintercalation produce heat, particularly during rapid charging or discharging cycles.
- Inefficiencies: No battery system is perfectly efficient. Hence, the remaining energy transforms into thermal energy.
The generated heat can potentially lead to several issues. Excessive heat can increase the risk of thermal runaway, where rising temperatures can cause further energy release, potentially leading to battery failure. Monitoring and managing this heat is critical, especially in applications such as electric vehicles and portable electronic devices.
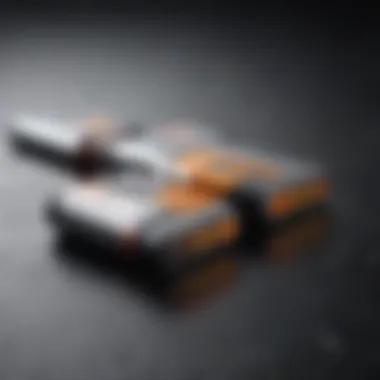
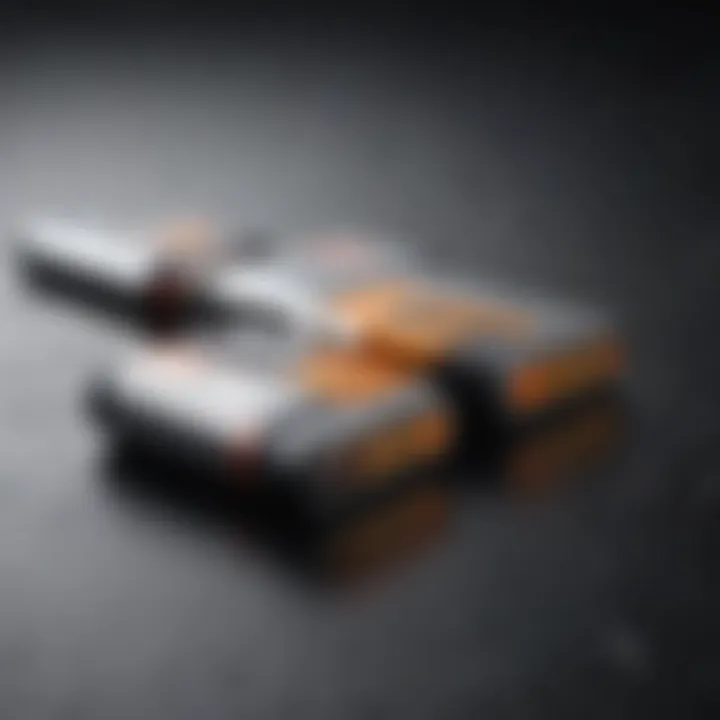
Impact on Battery Lifespan
The thermodynamic factors at play significantly influence the longevity of lithium ion batteries. High temperatures accelerate chemical reactions, often leading to faster degradation of materials. Some key points regarding the impact of heat on battery lifespan include:
- Accelerated Aging: Exposure to elevated temperatures can cause structural changes in the anode and cathode materials. This can reduce the number of charge cycles a battery can undergo before its capacity drops significantly.
- Electrolyte Decomposition: High temperatures may lead to undesirable reactions within the electrolyte, causing it to decompose and reducing overall battery performance.
- Cycle Efficiency Loss: Elevated temperatures can also result in reduced efficiency during charging and discharging cycles, leading to lower overall energy yield from the battery.
"Understanding how heat influences battery chemistry is essential for extending battery life and reliability."
Battery Efficiency and Performance Metrics
Battery efficiency and performance metrics are crucial in determining the real-world usability of lithium ion batteries. These aspects inform consumers and manufacturers about how well a battery performs under various conditions, including energy capacity, longevity, and environmental impact. Understanding these metrics enables stakeholders to make informed decisions in fields ranging from consumer electronics to electric vehicles.
Energy Density
Energy density refers to the amount of energy a battery can store relative to its size or weight. Higher energy density means that batteries can deliver more power without needing to increase in size, which is particularly important in applications like smartphones and electric vehicles. For lithium ion batteries, energy density typically ranges around 150 to 200 Wh/kg.
Several factors contribute to this performance metric:
- Material Composition: The choice of materials used in the anode and cathode directly affects energy density. For instance, lithium cobalt oxide offers higher energy density compared to lithium iron phosphate.
- Electrolyte Conductivity: A well-chosen electrolyte can improve the movement of lithium ions, thereby increasing efficiency and energy retention.
- Temperature Conditions: The environmental temperature can affect how efficiently a battery operates. Batteries tend to perform optimally within a certain temperature range.
The importance of energy density is accentuated in the context of sustainability, ensuring that devices and vehicles can operate longer on a single charge. Higher density reduces the frequency of charging cycles, which can extend battery life and lower the overall environmental footprint.
Cycle Life
Cycle life measures the number of complete charge and discharge cycles a lithium ion battery can undergo before its capacity fades significantly. A longer cycle life is favorable, as it means reduced waste and lower costs over time. Typical lithium ion batteries may achieve between 500 to 1000 cycles, depending largely on their chemistry and usage conditions.
Factors impacting cycle life include:
- Depth of Discharge: Regularly cycling a battery to its full discharge point can significantly reduce the overall cycle life.
- Charge Rate: Charging a battery too quickly can result in a higher level of stress on its components, leading to decreased longevity.
- Temperature Fluctuations: Excessive heat can deteriorate battery components faster, thus reducing cycle life.
In practical applications, improving cycle life is essential not only for cost efficiency but also for resource conservation. As the demand for batteries continues to rise, particularly in sustainable technology sectors, understanding and optimizing these performance metrics will remain a priority for innovation in lithium ion technology.
In summary, grasping the intricacies of battery efficiency and performance metrics, particularly energy density and cycle life, is essential for advancing technology while promoting sustainability. Understanding these metrics can lead to better designs and increased adoption of lithium ion solutions in various fields.
Challenges and Limitations in Lithium Ion Technology
Lithium ion batteries have become a cornerstone in modern energy storage solutions. However, they also face significant challenges and limitations that must be addressed to enhance their performance and safety. Understanding these aspects is crucial, as they significantly impact the reliability and adoption of lithium ion technology in various applications.
Safety Concerns
Safety is one of the paramount concerns regarding lithium ion batteries. Their complex chemistry and energy density can lead to severe incidents if not managed properly.
Thermal Runaway
Thermal runaway refers to a thermal reaction within a lithium ion battery that occurs when the battery temperature rises uncontrollably. This phenomenon often starts at a specific point, typically due to internal short circuits or external heat sources. As temperature increases, it can lead to a series of reactions releasing even more heat. This self-sustaining cycle can culminate in fires or even explosions.
The key characteristic of thermal runaway is its rapid escalation. Once this process begins, it can be nearly impossible to halt, making this aspect critical in battery management systems. The danger of thermal runaway highlights the need for adequate thermal management in battery design
Some advantages of understanding thermal runaway include improved safety measures in battery technology development. Manufacturers need to create batteries with better heat resistance and protective measures to mitigate this risk. However, the disadvantages include the added complexity and cost associated with such engineering solutions.
Battery Fires
Battery fires represent a severe manifestation of safety concerns with lithium ion batteries. When a battery experiences thermal runaway, it can ignite due to the flammable electrolyte and other materials within the cell.
The key characteristic of battery fires is their unpredictability. While rare, when they do occur, they can result in property damage and pose risks to human lives. The harmful smoke and toxic fumes released during such incidents compound the problem further.
The unique feature of battery fires is their potential for rapid spread, especially in devices where multiple batteries are used, such as electric vehicles. Understanding battery fire dynamics can lead to better designs that are less prone to such incidents. However, the disadvantages include increased scrutiny and regulation concerning battery use in consumer products.
Environmental Impact
The environmental impact of lithium ion batteries is another area of concern. While these batteries are crucial for reducing carbon emissions when used in electric vehicles, their production and disposal processes raise significant ecological issues.
Mining for lithium, cobalt, and other essential elements can result in habitat destruction and water depletion. The extraction processes also often involve chemicals that can pollute nearby ecosystems. Furthermore, once batteries reach the end of their lifecycle, improper disposal can lead to soil and water contamination.
Thus, there is a pressing need for sustainable practices in battery production and recycling efforts. Efforts to improve battery recycling are increasingly important as the number of batteries in use continues to rise globally. Effective recycling methods not only reduce environmental impact but also enhance resource efficiency by reclaiming valuable materials for reuse.
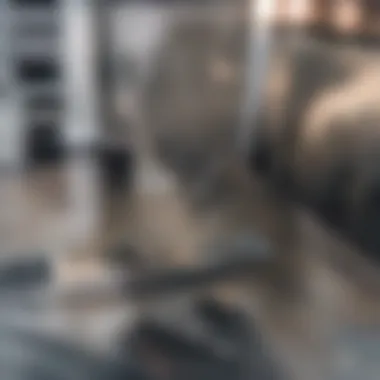
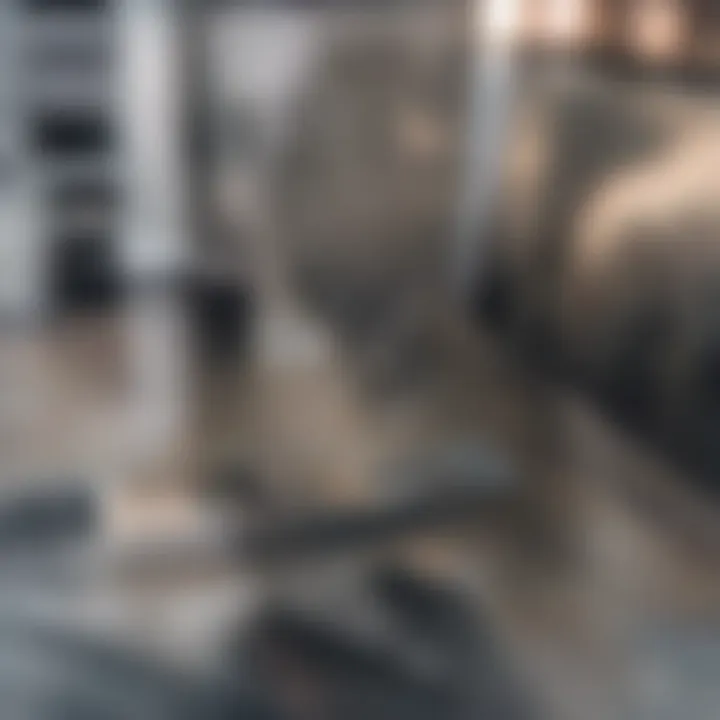
Overall, the challenges and limitations faced by lithium ion technology can significantly affect its future trajectory. Addressing these concerns is imperative for ensuring the safe, efficient, and sustainable use of lithium ion batteries in an increasingly energy-dependent world.
Recent Advancements in Battery Technology
Recent advancements in battery technology are pivotal in shaping the future of energy storage solutions. As the demand for efficient energy storage grows, significant innovations have emerged to enhance the performance, safety, and sustainability of lithium ion batteries. In this section, we will particularly focus on two important advancements: solid state batteries and the development of new anode and cathode materials.
Solid State Batteries
Solid state batteries represent a promising alternative to traditional lithium ion designs. They utilize a solid electrolyte instead of a liquid one, which can mitigate many of the safety issues associated with fluid electrolytes. This is crucial in reducing risks like thermal runaway and flammability.
The solid electrolyte allows for greater energy density, meaning that more energy can be stored in a smaller space. This has the potential to significantly increase the range of electric vehicles and the capacity of portable electronics.
Benefits of solid state technology include:
- Improved safety: The absence of volatile liquid electrolytes lowers the likelihood of battery fires.
- Enhanced lifespan: Solid state batteries typically endure more charge and discharge cycles without degradation.
- Higher energy density: The compact design supports greater energy storage, beneficial for various applications.
Nevertheless, challenges remain. These include production costs, scalability, and the need for new materials to facilitate performance. Companies like QuantumScape and Solid Power are exploring these issues, seeking to make solid state batteries a mainstream solution in the coming years.
New Anode and Cathode Materials
The pursuit of improved anode and cathode materials is another key advancement in lithium ion battery technology. Traditional graphite anodes are being challenged by alternatives such as silicon and lithium metal.
- Silicon Anodes: Silicon can theoretically provide much higher capacity than graphite. When silicon absorbs lithium ions, it undergoes significant expansion. This attribute, however, leads to mechanical stress and can cause the anode to break down over time. Overcoming this issue requires innovations in material engineering to create composite structures or coatings that can endure the stresses of cycling.
- Lithium Metal Cathodes: Lithium metal offers high energy density but also brings challenges related to dendrite formation. Dendrites are needle-like structures that can grow during charging, potentially leading to short circuits. Therefore, development efforts focus on designing effective separators and solid electrolytes that can prevent dendrite growth while maximizing performance.
Advancements in cathode materials are also noteworthy, especially with compounds such as lithium iron phosphate and layered nickel-cobalt-aluminum. These materials offer benefits like improved thermal stability and enhanced cycle life. Such innovations pave the way for more durable and efficient battery systems.
In summary, continuous research on solid state batteries and new materials for anodes and cathodes holds significant promise for the future. They are critical in addressing the limitations of traditional lithium ion batteries, thus contributing to safer, longer-lasting, and more efficient energy storage solutions.
Quote: "The future of energy storage hinges on the innovations in battery technology that can meet the increasing demands of modern applications."
These advancements are not only crucial for personal electronics but also for large-scale applications in electric vehicles and renewable energy systems. As these technologies mature, their potential to revolutionize energy storage becomes increasingly evident.
Future Directions in Lithium Ion Battery Research
The future directions in lithium ion battery research hold significant promise for improving battery efficiency, sustainability, and overall performance. As the demand for energy storage solutions grows, addressing the limitations of current technologies becomes crucial. Advancements in this field can directly impact renewable energy integration, electric vehicles, and portable electronics. Therefore, it is essential to explore areas like scalability, mass production, recycling, and sustainability to improve the lifecycle of lithium-ion batteries.
Scalability and Mass Production
In order to meet the increasing worldwide energy needs, it is essential that lithium-ion batteries can be mass-produced without compromising quality or safety. Scalability in manufacturing processes not only reduces costs but also facilitates widespread adoption of this technology across various sectors. Several approaches can be taken to enhance scalability:
- Automation and Robotics: Introducing automation in production lines maintains consistency and accuracy while reducing labor costs.
- Advanced Materials: Utilizing materials that are more readily available can lessen supply chain issues. For example, reducing dependence on cobalt through alternative chemistries can streamline production.
- Process Optimization: Improving manufacturing techniques, such as coating and processing, can significantly boost production efficiency.
By focusing on these elements, manufacturers can scale operations more effectively and help make lithium-ion batteries more accessible to consumers and businesses alike.
Recycling and Sustainability
As lithium-ion batteries become ubiquitous, the need for effective recycling and sustainability practices is paramount. Successful recycling protocols not only reduce waste but also recover valuable resources, contributing to a circular economy. Key considerations in enhancing recycling processes include:
- Closed-Loop Recycling: Developing methods for recycling that allow materials to be reused in new battery production can minimize the need for raw materials.
- Technological Innovation: Advancements in recycling technology can improve the rate and efficiency of material recovery. There is ongoing research to optimize the separation processes of lithium and other components to enhance yield.
- Consumer Awareness: Encouraging consumers to return used batteries through incentives or easy-to-access collection points can improve recycling rates.
Implementing robust recycling strategies will not only address environmental concerns associated with battery disposal but also ensure that material scarcity does not hinder the growth of lithium-ion technology.
Investing in recycling and sustainability is not only a prudent environmental choice but also a critical business strategy as lithium-ion batteries become more integrated into our daily lives.
End
In this article, we have explored the mechanisms of lithium ion batteries, emphasizing critical components and their operation. Recognizing the interplay between the anode, cathode, and electrolyte highlights its significance not only in technological advancement but also in energy sustainability. The efficiency and lifespan of these batteries are directly influenced by their design and material selection.
Summarizing Key Points
We have discussed the fundamental structure and principles governing lithium ion batteries. Key insights include:
- Charge and discharge processes: Lithium ions move between the anode and cathode, facilitating energy storage and release.
- Materials: The choice of materials affects battery performance, efficiency, and safety.
- Challenges: Issues such as thermal runaway and environmental impact pose risks that need to be addressed.
- Recent advancements: Innovations such as solid-state batteries and new materials offer promising directions for improving battery technology.
This summary reflects the complexity and importance of lithium ion battery mechanisms, delineating how they shape modern energy solutions.
The Future of Energy Storage
Looking ahead, the future of energy storage is closely tied to the evolution of lithium ion technology. Several factors will play critical roles:
- Scalability: Advancements in manufacturing processes will influence the mass production of batteries, reducing costs and making them more accessible.
- Recycling: Developing efficient recycling processes will mitigate environmental concerns and enhance sustainability.
- Emerging technologies: Innovations in battery chemistry and structure promise to yield more robust, long-lasting, and safer batteries.
Ultimately, the trajectory of lithium ion batteries will reflect our capacity to navigate challenges and harness opportunities, thereby shaping the landscape of energy storage for the foreseeable future.