Exploring Phototransistor Circuits and Their Applications
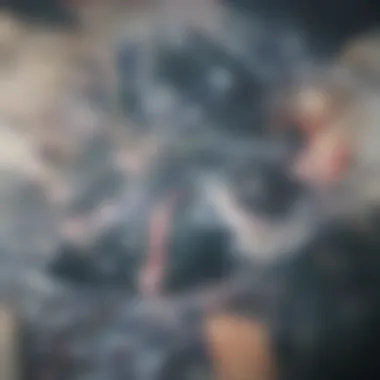
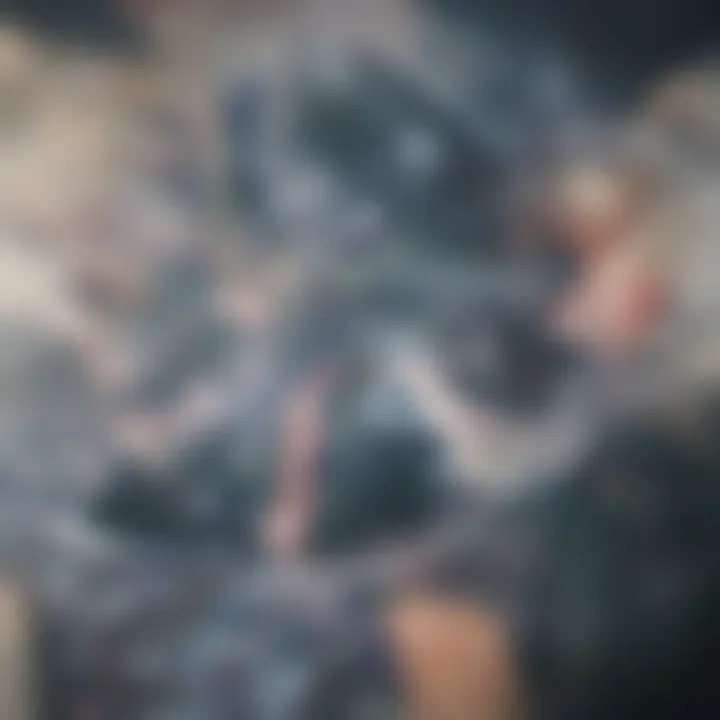
Intro
In the realm of electronics, phototransistors play a crucial role in various applications, from simple light detection systems to complex signal processing circuits. Understanding how these components function can provide insights into broader electronic principles. This article will dissect the components, operational principles, design considerations, and future outlook of phototransistor circuits, offering a comprehensive resource for those interested in the field.
Research Overview
Summary of Key Findings
Phototransistors are semiconductor devices that convert light into electrical signals. They function primarily through the interaction of light with their semiconductor material. The findings reveal that phototransistors are not only efficient in light detection but also versatile in their application. They can be found in consumer electronics, sensors, and communication devices.
Background and Context
The development of phototransistors began in the mid-20th century, coinciding with advancements in semiconductor technology. The ability to detect light and convert it into an electrical signal has paved the way for innovative applications in various fields such as automation, medical devices, and environmental monitoring. Their significance in technology continues to grow due to emerging applications in fields like IoT and smart home systems.
Key Components of Phototransistor Circuits
Phototransistor circuits are composed of several essential components:
- Phototransistor: The core component that responds to light.
- Resistors: Used for limiting current and setting the gain of the circuit.
- Capacitors: Provide stability and can filter noise from the signal.
- Power Supply: Required to power the entire circuit.
Understanding these components is vital for designing functional circuits that utilize phototransistors effectively.
Operating Principles
Phototransistors operate by absorbing photons, which causes charge carriers to be generated within the semiconductor material. This process leads to a change in the electrical current flowing through the device. The key operational aspects are:
- Light Sensitivity: The ability of the phototransistor to respond to varying light intensities.
- Response Time: The speed at which the phototransistor reacts to changes in light levels, critical in applications like pulse detection and communication systems.
Practical Designs
Practical designs of phototransistor circuits can take many forms. Common configurations include:
- Switching Circuits: Where the phototransistor acts as a switch, turning on and off based on light presence.
- Amplifier Circuits: Amplifying weak light signals for clearer detection.
Each design choice influences the effectiveness and reliability of the application.
Future Innovations
The evolution of phototransistor technology shows promise in addressing the growing demands of modern applications. Recent advancements include:
- Integration with Microcontrollers: Enhancing functionality in smart devices.
- Increased Sensitivity and Efficiency: Leading to new applications in low-light environments.
As the technology matures, the potential for new, groundbreaking applications continues to expand, encouraging exploration in various sectors.
"Phototransistor circuits represent an intersection of simplicity and sophistication in electronic design, making them timeless components in the evolving landscape of technology."
Prelude to Phototransistor Circuits
Phototransistor circuits play a crucial role in modern electronics by detecting light and converting it into electrical signals. Understanding these circuits is essential for electronics enthusiasts, students, and professionals. This area of study reveals the functionality behind numerous systems we interact with daily, such as optical communication devices and automated control systems.
Phototransistors serve as key components in various applications due to their ability to respond to light. They are a specialized type of transistor that can amplify current or switch electric signals based on light exposure. This unique characteristic opens the door to numerous applications. Importantly, comprehending how phototransistor circuits work can facilitate improved designs and innovations in the field.
There are several considerations when it comes to phototransistor circuits. These include understanding the physical structure of phototransistors, their operational principles, and their diverse applications. Grasping these concepts is vital for anyone aiming to design efficient circuits.
In summary, this section lays the groundwork for deeper exploration into the inner workings, types, and uses of phototransistor circuits. It not only defines what phototransistors are but also presents their historical journey and growing significance in technological advancement.
Definition and Role of Phototransistors
A phototransistor is a semiconductor device that converts light into electrical current. It functions similarly to a traditional transistor, but with the added capability of responding to light. When photons strike the phototransistor, they create electron-hole pairs within its material. This process allows the device to amplify current and detect light intensity, making it invaluable in applications ranging from digital communications to safety systems.
The role of phototransistors in circuits cannot be overstated. They are often used in sensors, switches, and amplifiers. For instance, in a simple light-sensing circuit, a phototransistor might automatically turn on a light when ambient light levels drop, showcasing its immediate practical benefits. Additionally, in telecommunications, phototransistors enable the rapid transmission of information through fiber-optic networks by converting light signals into electrical signals and vice versa.
Historical Development of Phototransistor Technology


The invention of the phototransistor traces back to the mid-20th century. Initially, the development of the transistor paved the way for other electronic innovations. In 1950, the first phototransistor was developed, demonstrating the potential of combining light-sensitivity with amplification properties. This event marked a significant milestone in electronic components.
Over the decades, advancements in materials and fabrication techniques have led to the miniaturization and enhancement of phototransistor technology. The introduction of new materials, such as gallium arsenide, expanded the functionalities of phototransistors. These developments greatly increased sensitivity and operational speed, aligning with the growing demands of electronic applications.
Today, phototransistors are integral to countless electronic devices, highlighting their evolution from simple light-sensitive elements to sophisticated components in complex systems. Understanding this history provides context for appreciating their current applications and future developments.
Fundamentals of Phototransistors
The understanding of phototransistors serves as the foundation for utilizing their unique properties in electronic applications. These devices convert light into electrical signals, making them indispensable in various fields, including optical communications, automation, and consumer electronics. Grasping the basics of phototransistor construction and operation opens up pathways for innovation and effective troubleshooting. Engineers and technicians often refer to these fundamentals to ensure optimal designs and implementations.
Construction of Phototransistors
Phototransistors have a specific architecture that differentiates them from conventional transistors. The basic structure typically comprises three layers: emitter, base, and collector. This layered composition allows phototransistors to function effectively under different lighting conditions.
- Epitaxial Layers: The layers are formed through epitaxy, which enhances the device's ability to detect light.
- Light-sensitive Material: Common materials include silicon, gallium arsenide, and indium gallium nitride. Selecting the suitable material impacts performance, responsiveness, and the spectral range of light that the device can effectively detect.
- Doping: Electrical characteristics are modified through doping processes. The amount and type of dopants used determine the conductivity of each layer and influence the efficiency of light absorption.
The interconnections of these layers determine the phototransistor's characteristics, such as gain and response time. Variations exist in design, including configurations like the planar phototransistor and surface-mounted devices.
Operational Principles of Phototransistors
When examining the operational principles of phototransistors, it is essential to understand how they convert light into electrical signals. At a basic level, phototransistors operate on the principle of generating charge carriers when exposed to light.
- Photoelectric Effect: When photons strike the junction of the phototransistor, they excite electrons, resulting in the creation of electron-hole pairs.
- Current Generation: The presence of these charge carriers leads to a flow of current. The efficiency of this process varies based on the intensity of light and the material characteristics.
- Amplification: Typically, phototransistors provide electrical gain. This means that even a small amount of incoming light can generate a substantially larger current at the output.
"Phototransistors represent a pivotal innovation in modern electronics, bridging the gap between photonic and electronic systems."
Phototransistors can operate in different modes. In active mode, they amplify the incoming light signals. On the other hand, in saturation mode, they switch from fully off to fully on in response to light. This binary operation is crucial for signal processing in digital circuits.
Types of Phototransistors
Understanding the various types of phototransistors is essential to grasping their applications and functionalities within circuits. Phototransistors serve as critical components in light detection and signal processing systems. Each type has distinct characteristics, making them suitable for different operational environments.
Bipolar Phototransistors
Bipolar phototransistors are one of the most common types of phototransistors. They utilize both electron and hole charge carriers, which makes them highly efficient in detecting light. When light strikes the phototransistor, it generates a photocurrent that is effectively amplified. This amplification is crucial because it allows for the detection of weak light signals in various applications.
A defining feature of bipolar phototransistors is their ability to operate in different configurations, such as common emitter and common collector. This versatility enables designers to optimize circuit performance, depending on specific use cases.
Some considerations for using bipolar phototransistors include their relatively slower response time compared to other types. Despite this, they remain prevalent in applications that require reliability and stability. Here are some key applications:
- Light detection in alarm systems
- Ambient light sensing in consumer devices
- Light-to-voltage conversion in solar panels
Photosensitive Field Effect Transistors
Photosensitive field effect transistors, often abbreviated as photosensitive FETs, operate fundamentally differently than bipolar phototransistors. These components leverage electric fields to control current flow, making them suitable for integrated circuits and high-speed applications.
Unlike bipolar types, photosensitive FETs typically exhibit faster response times, which is beneficial in scenarios where time-sensitive detection is critical. Their structure allows them to be integrated directly into semiconductor substrates, resulting in smaller devices with potentially lower costs.
However, careful selection is imperative because their sensitivity to light conditions can vary based on the material used and design architecture. Applications of photosensitive FETs include:
- High-frequency optical communication
- Smart home automation systems
- Imaging applications in cameras
Effective design and configuration can significantly enhance the performance of both bipolar phototransistors and photosensitive FETs in practical circuit implementations.
In summary, both bipolar phototransistors and photosensitive field effect transistors play pivotal roles in the realm of light sensing technologies. Their unique benefits come into play across various applications, offering solutions tailored to specific requirements.
Phototransistor Circuit Configurations
Phototransistor circuit configurations play a crucial role in leveraging the operational capabilities of phototransistors effectively. Understanding these configurations allows engineers and designers to select the appropriate setup for specific applications. By doing so, they can enhance performance, increase efficiency, and ensure reliability in various light-sensitive tasks. Each configuration has unique characteristics tailored to different functionalities, ranging from signal amplification to precise switching operations. The benefits and considerations associated with these configurations are essential for both novice and experienced individuals in the electronics field.
Basic Phototransistor Amplifier Circuits
Basic phototransistor amplifier circuits are fundamental in enhancing weak signals generated by light exposure. These circuits take advantage of the light-sensitive characteristics of phototransistors, converting light into electrical signals that can be amplified for further processing. Typically, these circuits involve a phototransistor connected to a load resistor. When light hits the phototransistor, it generates a current proportional to the light intensity, which causes a voltage drop across the resistor, resulting in an amplified output.
Key considerations for designing basic amplifier circuits include:
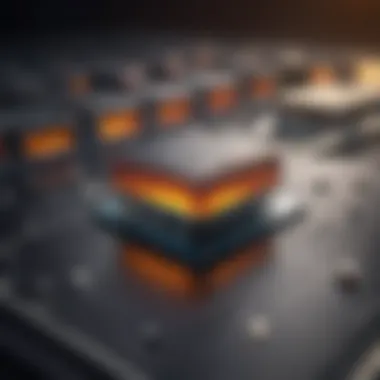
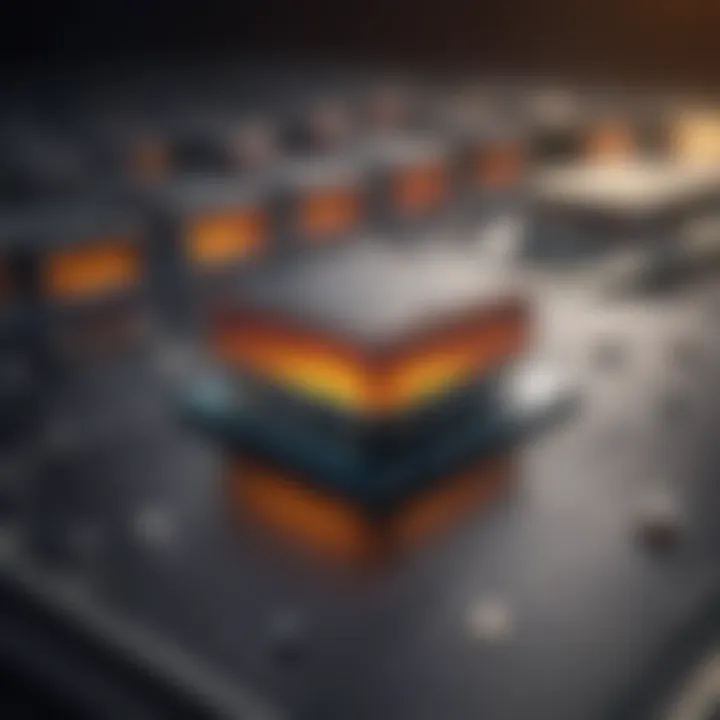
- Gain requirements: Understanding how much amplification is needed for the application.
- Load conditions: Assessing the load the circuit must drive to ensure proper function.
- Signal-to-noise ratio: Maintaining a high ratio for clean output.
In practice, using specific configurations, such as common-emitter or common-collector setups, can lead to different amplifier characteristics.
Phototransistor Switching Circuits
Phototransistor switching circuits utilize phototransistors as electronic switches that can be actuated by incident light. These circuits are particularly advantageous in applications where physical switches may not be feasible. A phototransistor acting as a switch can provide isolation between the control side and the load side, enhancing safety and operational stability.
A common configuration involves placing the phototransistor in series with a load. When light reaches the phototransistor, it turns on, allowing current to flow through the load. This simple configuration can be expanded to include additional functions such as:
- Timing controls: Creating delays before switching.
- Logical operations: Combining multiple switches for complex functions.
- Proximity sensing: Detecting the presence of objects based on reflected light.
Designing effective phototransistor switching circuits requires understanding the switching speed and saturation characteristics, helping to minimize latency and maximize performance.
Analog versus Digital Phototransistor Circuits
The distinction between analog and digital phototransistor circuits is significant in determining their applications and functionality. Analog circuits involve continuous signal processing, where the phototransistor generates varying output based on light intensity. These circuits are optimal for applications requiring nuanced responses to different light levels, such as automatic brightness control in displays.
In contrast, digital circuits utilize phototransistors to create binary outputs, typically functioning as on/off switches. This configuration is useful in environments where specific light thresholds indicate distinct operational states. Employing analog phototransistors provides:
- Greater sensitivity to light variations.
- Smooth signal transitions, ideal for controlling devices with gradual responsiveness.
While digital configurations offer:
- Simpler design and implementation, given the binary nature.
- Reduced noise interference, enhancing reliability in digital systems.
Analyzing the requirements of a project will dictate the appropriate choice between analog and digital phototransistor circuits. By discerning these differences, engineers can improve the performance and effectiveness of their designs.
Applications of Phototransistor Circuits
Phototransistors are central components in various applications where light detection and response are crucial. Understanding these applications provides insights into how phototransistor circuits are utilized in real-world scenarios. The implications of their use extend from enhancing communication systems to improving automation processes.
The importance of exploring the applications of phototransistor circuits stems from their versatility and functionality in different fields. By adapting to light, phototransistors serve various roles, positioning them as a pivotal technology in modern electronics. Here, we analyze three primary areas of application.
Optical Communication Systems
In optical communication systems, phototransistors play a vital role by converting light signals into electrical signals. This conversion is essential for transmitting information over distances using light waves, particularly in fiber optics. The ability of phototransistors to operate at high speeds allows for the transmission of large amounts of data efficiently.
For example, in telecommunications, phototransistors can detect light pulse signals, which represent digital data. Their effectiveness in signal detection directly impacts the clarity and speed of communication.
Key benefits include:
- High-speed data transfer capabilities.
- Enhanced signal integrity due to minimized noise interference.
- Compatibility with various wavelengths of light, enabling broader application.
Industrial Automation and Control
Phototransistors are also widely used in industrial automation and control systems. They serve as sensors that detect light to control machinery or measure environmental conditions. These sensors can activate switches based on specific light thresholds, making them efficient for systems that require automatic responses to changing conditions.
For instance, in automatic assembly lines, phototransistors are integral in safety systems. They detect the presence or absence of products on the line, allowing machines to operate pertinent controls effectively. Other considerations in this application include:
- Reducing human error through automation.
- Enhancing operational efficiency by improving response times.
- Diminishing the risk of accidents in hazardous environments.
Consumer Electronics
In the realm of consumer electronics, phototransistors find applications in devices like remote controls, cameras, and light meters. They are instrumental in light-sensitive operations. For example, in digital cameras, phototransistors help in autofocus systems by measuring the intensity of light reflected from the subject.
Similarly, devices like infrared remote controls use phototransistors to receive signals, allowing for seamless communication between the remote and the device itself. This has direct implications in user convenience and device efficiency. Benefits of this application include:
- Enhanced functionality of electronic devices.
- Increased user accessibility and control.
- Improved response to varying lighting conditions.
In summary, the applications of phototransistor circuits are diverse and impactful across numerous fields. Their adaptability and efficacy make them indispensable in modern technology. Understanding these applications not only reveals their contribution to existing technologies but also indicates their potential for future innovations.
"Phototransistors are essential in bridging the gap between light signals and electronic processing, shaping the future of communication and control systems."
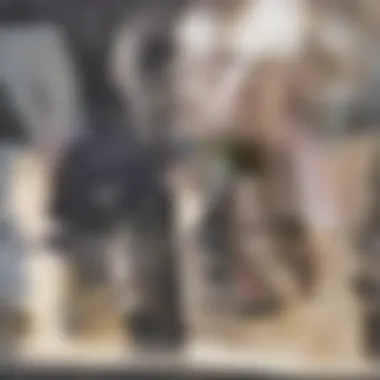
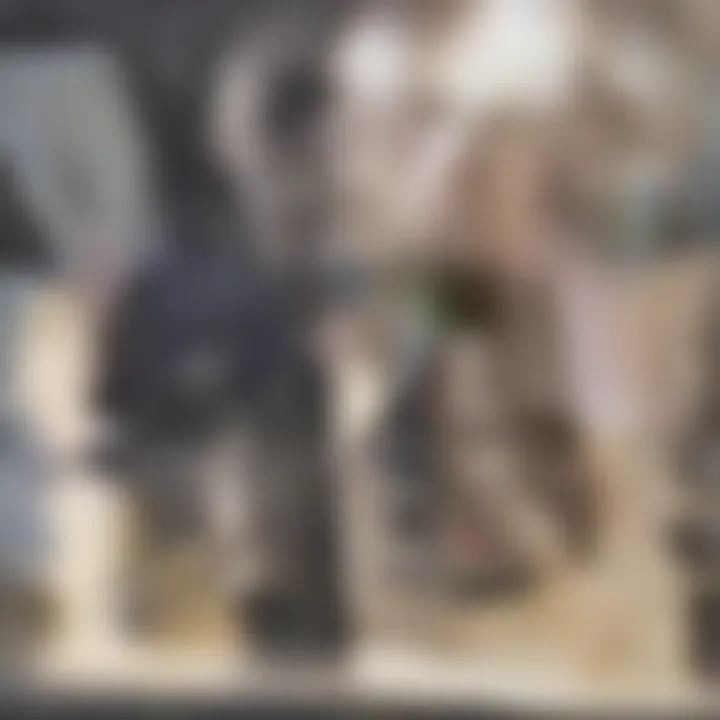
These intricacies underline the significance of mastering phototransistor circuits for students, researchers, educators, and professionals alike.
Design Considerations for Phototransistor Circuits
Designing effective phototransistor circuits involves several crucial considerations. These elements play a key role in ensuring the performance and reliability of the circuits. Understanding these factors is essential for students, researchers, educators, and professionals who work with phototransistors in various applications. The main focus will be on selecting the right phototransistor and determining circuit parameters for optimal operation.
Selecting the Right Phototransistor
Choosing the appropriate phototransistor is vital for any circuit design. The selection process involves several criteria that impact performance. Key aspects to consider include:
- Type of Phototransistor: Whether to use a bipolar or photosensitive field-effect transistor affects the current and voltage characteristics. Each type has distinct advantages based on the application.
- Wavelength Sensitivity: Different phototransistors respond to various light wavelengths. Depending on the use case, select a phototransistor that aligns with the required light spectrum.
- Response Time and Gain: The speed at which a phototransistor generates a signal and the amplification factor are critical in high-speed applications. Analyzing these parameters ensures the device meets the demands of the project.
- Operating Voltage and Current Specifications: Ensure that the chosen phototransistor can operate within the voltage range and current levels specific to your circuit. Exceeding these values can lead to device failure.
In summary, selecting the right phototransistor involves a careful assessment of type, sensitivity, speed, and specifications. This decision sets the foundation for engineered circuits that function correctly and efficiently.
Determining Circuit Parameters
Once the phototransistor is selected, determining the circuit parameters is the next crucial step. This consideration involves understanding how various components interact in the circuit. Important parameters include:
- Load Resistance: The resistance value directly influences the current flow and voltage drop across the phototransistor. Calculate optimal load resistance to enhance system performance, aligning with the desired output.
- Biasing Circuits: Proper biasing of the phototransistor ensures it operates in the correct region. Calculate the biasing conditions that sustain the operating regions for reliable performance when exposed to light.
- Signal Conditioning: Depending on the application, additional components like amplifiers and filters may be required to refine signal quality. When determining circuit parameters, plan for these additions to avoid issues with noise or interference.
- Temperature Effects: Phototransistors' performance can vary with temperature changes. Understanding how the circuit reacts in different thermal environments ensures circuit stability and reliability.
By effectively determining these circuit parameters, one can optimize phototransistor circuits for specific applications. It tailors the design to meet performance standards while accommodating future requirements.
"Effective design considers both component selection and circuit behavior, leading to robust and reliable phototransistor applications."
With these considerations addressed, engineers and designers can build circuits that not only function correctly but also excel in their application scenarios.
Troubleshooting Phototransistor Circuits
In the realm of phototransistor circuits, troubleshooting is a critical aspect that ensures circuits operate effectively. Understanding how to diagnose and resolve faults within these circuits is essential for maintaining functionality and reliability. Given the intricate nature of phototransistors, both in design and application, recognizing the importance of troubleshooting can prevent extensive downtime and enhance the performance of electronic systems. It is also relevant for professionals who frequently work with optical devices, as effective troubleshooting techniques can lead to quicker repairs and enhanced system reliability.
Common Faults and Their Diagnosis
When dealing with phototransistor circuits, several common faults can arise. Each fault manifests uniquely, necessitating a keen understanding of how to identify them. Some of the most prevalent issues include:
- Light Exposure Problems: A common issue occurs when the phototransistor fails to respond to light stimuli. This can be due to a blocked sensor or incorrect positioning within the circuit.
- False Triggering: Sometimes, the phototransistor might trigger without actual light input, often caused by noise or nearby electromagnetic interference.
- Component Failures: Variations in the circuitβs components, including resistors, capacitors, and even the phototransistor, can cause overall circuit inefficiency.
- Thermal Effects: High temperatures can adversely affect the performance of phototransistors. Understanding the thermal limits of components is crucial for effective troubleshooting.
- Wiring Errors: Incorrect wiring can lead to operational discrepancies. Wire shorts or breaks often impede circuit functionality.
Diagnosis of these faults typically involves methodical processes, such as:
- Visual Inspection: Begin with a careful examination of the circuit to spot any visible damage or loose connections.
- Use of Multimeter: A multimeter can help assess the voltage across various points in the circuit, identifying low or absent signals.
- Signal Testing: Monitoring the output signal when exposed to light can help identify discrepancies in operational response.
This systematic approach to diagnosing issues can significantly simplify the troubleshooting process, leading to quicker resolution of faults.
Repair Techniques and Solutions
Once faults have been identified within a phototransistor circuit, the next step involves applying effective repair techniques. Some common solutions include:
- Replacing Faulty Components: When a component such as the phototransistor itself is determined to be defective, replacing it with a new or tested equivalent becomes necessary. Ensure the replacement matches the specifications of the original component to maintain circuit integrity.
- Rewiring: For wiring errors, patiently tracing and correcting the wiring paths mitigates issues related to shorts or breaks.
- Adjustments to Positioning: If the phototransistor is inaccurately placed, reconfiguring its orientation or distance from light sources can enhance performance reliability.
- Filtering Noise: In cases of false triggering, integrating noise filters or isolating the circuit from electromagnetic interference often stabilizes operation.
- Implementing Heat Management: Introducing heatsinks or choosing temperature-resistant components aids in optimizing performance under thermal stresses.
In summary, the effective troubleshooting of phototransistor circuits encompasses identifying common faults and applying targeted repair techniques. By comprehensively understanding these processes, professionals can ensure the continuous functionality of their electronic systems.
Future Trends in Phototransistor Technology
The exploration of phototransistor technology is crucial in understanding the evolving landscape of electronics. This section focuses on what lies ahead, examining the trends that will shape the future of phototransistor circuits. Advancements in technology, particularly in material science and integration methods, are pivotal. These trends highlight both the potential benefits and new considerations for researchers and professionals.
Advancements in Material Science
One of the key areas of growth in phototransistor technology is material science. Research is ongoing into new semiconductors that can improve sensitivity and efficiency. For example, materials such as organic semiconductors and perovskites are gaining attention. These materials exhibit properties that could lead to better performance in low-light conditions. Additionally, they are often more cost-effective than traditional silicon-based materials.
Recent studies suggest that the development of these materials will permit the miniaturization of phototransistors without losing performance. This can lead to applications in compact electronic devices, such as smartphones and wearables. Further work is needed to enhance the stability and durability of these materials under various environmental conditions.
"The continual advancement in material technology is likely to redefine the effectiveness of phototransistors in numerous applications."
Integration with Other Technologies
As phototransistors evolve, their integration with other technologies becomes increasingly important. This trend is evident in the rise of smart devices and the Internet of Things (IoT). Phototransistors can be paired with sensors and microcontrollers, enabling a wide range of applications, from smart lighting to automated environmental controls.
The integration process often involves developing standardized protocols to ensure compatibility across devices. Moreover, collaboration with artificial intelligence technologies is on the rise. This combination allows for enhanced data processing and decision-making capabilities, making systems more efficient.
Potential future applications may include advanced security systems that utilize phototransistors for motion detection and smart home systems that can adapt to user preferences based on light conditions.
In summary, the future of phototransistor technology lies in innovative material developments and enhanced integration capabilities. These trends will not only increase the versatility of phototransistors but also expand their application scope significantly. Students, researchers, and professionals should pay close attention to these developments as they can greatly impact real-world applications.