Applications of SERS Nanoparticles in Modern Science
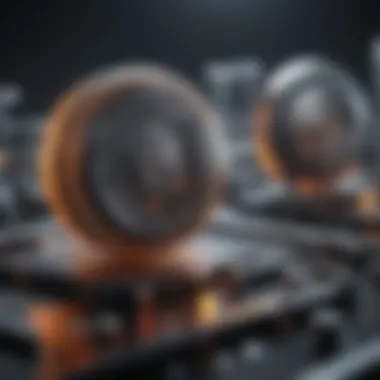
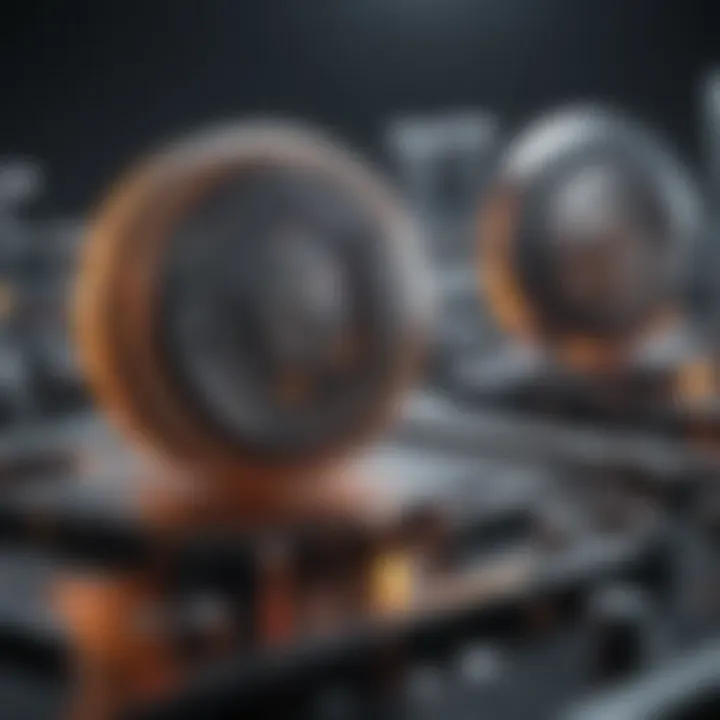
Intro
In the realm of modern science, surface-enhanced Raman scattering (SERS) nanoparticles are playing a pivotal role that cannot be overlooked. By amplifying the signals from molecules, these particles have opened new frontiers in disciplines ranging from molecular biology to environmental monitoring. In essence, SERS nanoparticles serve as a bridge between the minuscule world of molecules and the larger scientific narratives we strive to understand.
The fuss around SERS nanoparticles stems not just from their mechanism but from the wealth of applicative potential they bring. Picture this: By simply introducing a few nanoparticles to a sample, scientists can obtain vivid and insightful spectra that were once elusive. This method not only enhances the visibility of low-concentration substances but also provides a fine lens into complex biological systems, chemical interactions, and environmental changes.
As we delve deeper into the multifaceted applications of SERS nanoparticles, it is important to unpack their fundamental characteristics, the mechanisms that govern their efficacy, and the extensive array of uses that straddle various scientific fields. Each application reveals a glimpse into the profound possibilities held by these little wonders.
Research Overview
Summary of Key Findings
The research surrounding SERS nanoparticles has produced a treasure trove of findings that speak volumes about their capabilities. Recent studies highlight how these nanoparticles:
- Enhance Raman signals significantly, improving molecular detection limits.
- Can be tailored to specific applications with various materials and coatings, thus enabling their use in diverse environments.
- Act as vital tools in biosensing, allowing for the detection of diseases at early stages through biomarker identification.
- Provide insight into environmental pollutants at trace levels, thus serving as an early warning sign in ecological studies.
Background and Context
To understand the potential and the impact of SERS nanoparticles, one must start with the basic premise of Raman scattering itself. This phenomenon leverages the interaction of light with molecular vibrations—essentially, it’s a way to discern the ‘fingerprints’ of molecules. However, without the enhancement provided by nanoparticles, this technique often falls short for many applications. That's where SERS comes in.
The interplay between light and nanostructures, often metallic like silver or gold, amplifies the signals to a degree that it turns once challenging detections into something manageable and insightful. Over the last two decades, the field has rapidly evolved, positioning SERS nanoparticles as essential tools across various scientific inquiries.
The ongoing research focuses not only on refining the synthesis of these nanoparticles but also exploring innovative applications in healthcare, food safety, environmental monitoring, and beyond. The potential is staggering, marking SERS nanoparticles as a cornerstone of modern scientific advancements.
Preface to SERS Nanoparticles
Understanding SERS nanoparticles is crucial, not just for the specialists in nanotechnology but for anyone involved in modern scientific research. These tiny particles, with their remarkable ability to amplify the scattering signal of molecules, serve as a bridge connecting various fields—biology, chemistry, and environmental science, to name a few. Through this introduction, we’ll explore what SERS nanoparticles are and why they’ve become indispensable tools in numerous applications.
Definition and Significance
Surface-enhanced Raman scattering (SERS) nanoparticles are often described as futuristic tools that facilitate chemical detection and characterization at minute scales. At their core, SERS nanoparticles effectively enhance the Raman scattering of molecules adsorbed onto their surface. This phenomenon is significant because it allows for the identification of substances at extraordinarily low concentrations, rendering it beneficial for areas like biomedical diagnostics, environmental monitoring, and material science.
The importance of SERS nanoparticles is evident when we consider their incredible sensitivity. Traditional methods of chemical analysis might miss certain analytes, but with SERS, even a few molecules can be detected. This capability not only improves the reliability of experimental processes but also opens new avenues for research. For instance, the ability to detect biomarkers in bodily fluids can lead to early diagnosis of diseases, fundamentally changing how we approach health care.
Despite their small size, which is often measured in nanometers, the impact of SERS nanoparticles is anything but small. Their significance can't be overstated; they serve as powerful enablers of knowledge, revolutionizing what we can detect and analyze across numerous fields.
Historical Context
The journey of SERS nanoparticles is rooted deep in the history of scientific discovery. The background of SERS can be traced back to the 1970s when researchers first observed the remarkable enhancement of Raman signals on metal surfaces. The initial exploration was rather rudimentary but sparked curiosity in the connection between nanostructures and their scattering properties.
By the 1990s, advancements in nanofabrication techniques led to a significant evolution of SERS technology. Scientists observed that the multi-faceted geometric shapes of nanoparticles—such as gold and silver spheres, rods, and stars—provided diverse enhancement factors, making SERS more versatile than ever. These breakthroughs caught the attention of various scientific disciplines, who began to explore practical applications in diagnostics, environmental sensing, and even security.
As the 21st century dawned, the focus shifted further towards enhancing stability and reproducibility of SERS signals, leading to refined techniques in nanoparticle synthesis and functionalization. Today, the landscape is vastly different; SERS nanoparticles are now pivotal in cutting-edge research, with applications spanning from targeted drug delivery to real-time environmental monitoring.
In summary, understanding the definition and historical context of SERS nanoparticles is key to appreciating their transformative potential in modern science. Through continuous research, SERS technology is unveiling new possibilities that were once considered beyond reach.
Mechanisms of Surface Enhancement
Understanding the mechanisms of surface enhancement in SERS nanoparticles is essential in appreciating their role in various scientific realms. These mechanisms are the backbone of how SERS technology achieves its remarkable sensitivity, allowing detection of molecules at incredibly low concentrations. Not delving into these mechanisms would be akin to analyzing a masterpiece without understanding the strokes of the brush; they offer insight into the underlying processes that yield such great results.
In essence, SERS relies on two fundamental mechanisms—electromagnetic enhancement and chemical enhancement. Each plays a significant part in amplifying the Raman scattering signal of molecules adsorbed onto metallic surfaces. The interplay between these mechanisms contributes to the overall effectiveness of SERS in both qualitative and quantitative analysis.
Electromagnetic Mechanism
The electromagnetic mechanism is arguably the more predominant of the two when discussing surface enhancement. It operates on the premise that the interaction of light with metallic nanoparticles generates localized surface plasmons—collective oscillations of electrons at the surface of the nanoparticles. This means that when light hits a nanoparticle, it creates an intense electromagnetic field that significantly amplifies the Raman signal from nearby molecules.
Consider a simple analogy: imagine a room where a loudspeaker is pumping out tunes, and the vibrations cause the air molecules to sway. Now, if you placed a tiny microphone near that loudspeaker, it would pick up sound waves much more efficiently due to the proximity to the source. In a similar vein, molecules close to these plasmonic structures experience a boost in the intensity of Raman scattering, leading to enhanced signals.
This electromagnetic mechanism is heavily influenced by various factors: the size, shape, and material of the nanoparticles, as well as the wavelength of the incident light. For instance, silver and gold nanoparticles are typically preferred due to their favorable plasmonic properties.
Chemical Mechanism
In contrast, the chemical mechanism accounts for another layer of enhancement which, while lesser in magnitude, is not to be overlooked. This mechanism involves charge transfer interactions between the analyte molecules and the nanoparticle surface. When a molecule adheres to a metallic surface, there can be an electronic coupling between the two that leads to an increased probability of Raman scattering.
Imagine a dance where the participants share connection with each other, leading to more dynamic movements. In the SERS context, this coupling results in a more significant energy transfer, enabling a boosted Raman signal even without the strong electromagnetic field. The extent of this enhancement is contingent upon the nature of the molecular interaction. Molecules that have functional groups capable of forming bonds with the metal can exhibit enhanced signals through this chemical mechanism.
Role of Nanoparticle Morphology
Another critical aspect influencing SERS effectiveness is the morphology of the nanoparticles. The term "morphology" refers to the shape, size, and structural characteristics of the nanoparticles. It has been shown that specific structures, such as nanorods, triangular prisms, and nanoshells, can exhibit varying degrees of enhancement due to their unique plasmonic behavior.
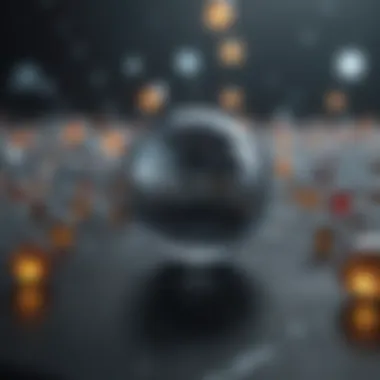
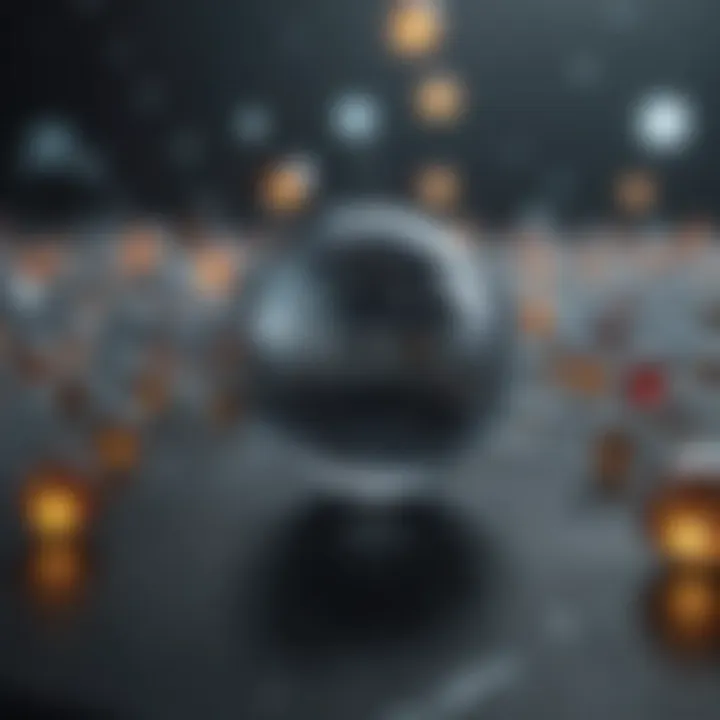
The arrangement of these nanoparticles also plays a pivotal role. Close-packed structures can create hotspots—sites where the electromagnetic field is significantly intensified. These truly are the sweet spots for SERS, where the combined effect of the electromagnetic and chemical mechanisms can lead to extraordinary detection capabilities.
"The design of nanoparticles with precise morphology can be the difference between a barely-there signal and a crystal-clear reading in a SERS experiment."
In summary, the mechanisms of surface enhancement, driven by both electromagnetic and chemical factors, combined with careful consideration of nanoparticle morphology, contribute significantly to the success of SERS technology. Understanding these intricacies enables scientists to innovate and push the boundaries of what SERS nanoparticles can achieve in practical applications.
Synthesis of SERS Nanoparticles
The synthesis of SERS nanoparticles serves as a cornerstone in understanding their applications and capabilities across various scientific fields. Without quality synthesis, the effectiveness of these nanoparticles in enhancing surface techniques like Raman scattering could be compromised. The ability to control properties such as size, shape, and surface chemistry directly impacts their performance, making synthesis a key focus area in SERS research. Factors like suitable materials and advanced fabrication techniques determine the extent to which these nanoparticles can amplify signals, thus bearing a pivotal role in ongoing innovations.
Top-Down versus Bottom-Up Approaches
Synthesis methods generally fall into two categories: top-down and bottom-up approaches. Each has its own merits and demerits in terms of scalability, cost, and complexity.
- Top-Down Approaches: This method involves breaking down bulk materials to form nanoparticles. Techniques like lithography and milling are commonly employed to achieve the desired sizes. While this approach can yield uniform particles, the process often requires intricate machinery and can be limited in terms of material variety.
- Bottom-Up Approaches: Contrary to top-down, this method constructs nanoparticles atom by atom or molecule by molecule. Chemical vapor deposition and sol-gel processes fall under this category. Although more flexible with material usage and scalability, bottom-up methods can sometimes lead to inconsistencies in size and distribution.
Ultimately, the choice of approach may depend on the intended application of the SERS nanoparticles, highlighting the need for a tailored synthesis strategy that fits specific scientific demands.
Common Materials Used
In the realm of SERS nanoparticle synthesis, material selection is crucial. Commonly used materials include:
- Gold (Au): Known for its excellent plasmonic properties, gold nanoparticles are widely employed due to their stability and strong SERS signal enhancement.
- Silver (Ag): Silver is often favored for its superior enhancement of Raman signals compared to gold, though it tends to be less stable in certain environments.
- Copper (Cu): Although not as common, copper nanoparticles are gaining traction due to their lower cost and comparable enhancement properties.
The choice of material not only affects the performance of the nanoparticles but also influences biocompatibility and environmental impact, which researchers are increasingly considering in their designs.
Functionalization and Modification Techniques
Functionalization plays a key role in optimizing SERS nanoparticles for specific applications. By modifying the surface chemistry, researchers can enhance stability, biocompatibility, and target functionality. Common functionalization techniques include:
- Covalent Bonding: This method attaches biomolecules or other reagents directly to the nanoparticle surface, improving specificity in sensing applications.
- Physical Adsorption: Through van der Waals forces, agents can be adsorbed onto the nanoparticle surface without forming strong bonds, offering a reversible approach for targeting purposes.
- Polymers and Coatings: Employing polymers enhances the stability and dispersibility of nanoparticles in biological environments, making them more effective in practical applications.
The efficiency of SERS nanoparticles heavily depends on their functionalization, which can lead to groundbreaking advancements in fields ranging from biomedical diagnostics to environmental monitoring.
In summary, the synthesis of SERS nanoparticles is a multi-faceted process that includes various approaches, materials, and modification techniques tailored for specific applications. Understanding these elements is essential for utilizing SERS nanoparticles effectively in both research and practical realms.
Characterization of SERS Nanoparticles
Characterization of SERS nanoparticles is crucial to harnessing their potential in various scientific and technological domains. At its core, the accurate characterization enables researchers to understand the specific properties of these nanoparticles that contribute to their enhanced functionality in surface-enhanced Raman scattering (SERS). It includes assessing factors such as size, shape, and surface chemistry, which all significantly influence the performance of SERS techniques in both fundamental research and application. Without a thorough understanding of these characteristics, it’s akin to navigating a ship without a compass; one may easily drift off course.
Well-characterized SERS nanoparticles lead to improved sensitivity, selective detection of analytes, and enhanced reproducibility in measurement, which are key aspects in the practical implementation of SERS technology. Moreover, they also pave the way for innovative applications in other fields like environmental monitoring and biotechnology. As such, characterization isn't just an academic exercise but serves as the backbone for advancing SERS applications.
Morphological Analysis
When diving into the morphological analysis of SERS nanoparticles, one must first appreciate that the shape and size of these nanoparticles have an enormous impact on how effectively they can enhance Raman signals. Different geometries—like spheres, rods, and triangles—affect the electromagnetic field interactions at the nanoparticle surface. For instance, gold and silver nanoparticles typically display heightened SERS capabilities due to their propensity for localized surface plasmon resonance (LSPR).
Microscopy techniques such as Transmission Electron Microscopy (TEM) and Scanning Electron Microscopy (SEM) are often employed to determine these morphological features. By visually scrutinizing the nanoparticles, researchers can correlate their observed properties with SERS performance. This correlation underscores the importance of tailoring nanoparticle morphology for specific applications—certain shapes can be more effective at enhancing signals from particular analytes.
Spectroscopic Techniques
Spectroscopic techniques form the bedrock upon which SERS characterization stands. One of the most significant methods is Raman spectroscopy itself, which, when coupled with SERS, becomes a powerful tool for analyzing the vibrational modes of molecules adsorbed onto the nanoparticle surfaces. This coupling not only enhances signal detection but allows for more specific and sensitive identification of various chemical compounds.
Moreover, other spectroscopic methods, such as UV-Vis spectroscopy, can offer insights into the optical properties of the nanoparticles, including their absorption and scattering behavior. By using these techniques, scientists can extract valuable parameters like excitation wavelength ranges and SERS enhancement factors, which are fundamental in optimizing SERS conditions. A thorough spectroscopic analysis thus ensures that the nanoparticles will perform as expected in real-world applications, providing a roadmap for future adjustments or advancements.
Nanoparticle Stability and Reproducibility
Evaluating nanoparticle stability and reproducibility is essential for ensuring that SERS applications yield consistent results. Nanoparticle stability refers to how well these particles maintain their structural integrity and functionality over time, while reproducibility encompasses the ability to achieve the same results across different samples and experiments.
Factors like environmental conditions, solvent effects, and even chemical interactions can influence both stability and reproducibility. Testing under a variety of conditions helps researchers understand these influences. For instance, it’s found that gold nanoparticles often have better stability in acidic conditions compared to silver nanoparticles, which might oxidize more readily.
To reinforce both these aspects, incorporation of stabilizing agents and surface coatings can be beneficial, ensuring that the nanoparticles not only retain their physical properties but also provide reliable SERS performance across various environments.
In summary, stability and reproducibility checkmarks are like the golden stars on report cards for scientists working with SERS nanoparticles; they signal the credibility and reliability of research findings to the broader scientific community.
"The future of SERS applications rests on our ability to finely characterize and standardize nanoparticle synthesis and behavior."
Thus, by focusing on these elements of characterization, the full breadth of SERS capabilities can be tapped into—a vital step for the ongoing advancement of this transformative technology.
Applications in Biological Systems
Applications of SERS nanoparticles in biological systems illustrate not only the adaptability of these tools but also their significant potential in transforming health sciences and biomedical research. With the ability to enhance Raman signals, SERS offers a profound avenue for analyzing biological substances with precision that traditional methods often lack. By leveraging the unique properties of nanoparticles, researchers are making strides in various domains of medicine, particularly in diagnostics, imaging, and therapeutics.
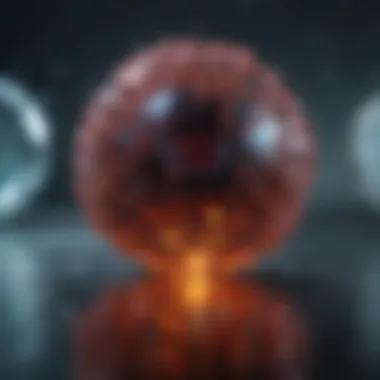
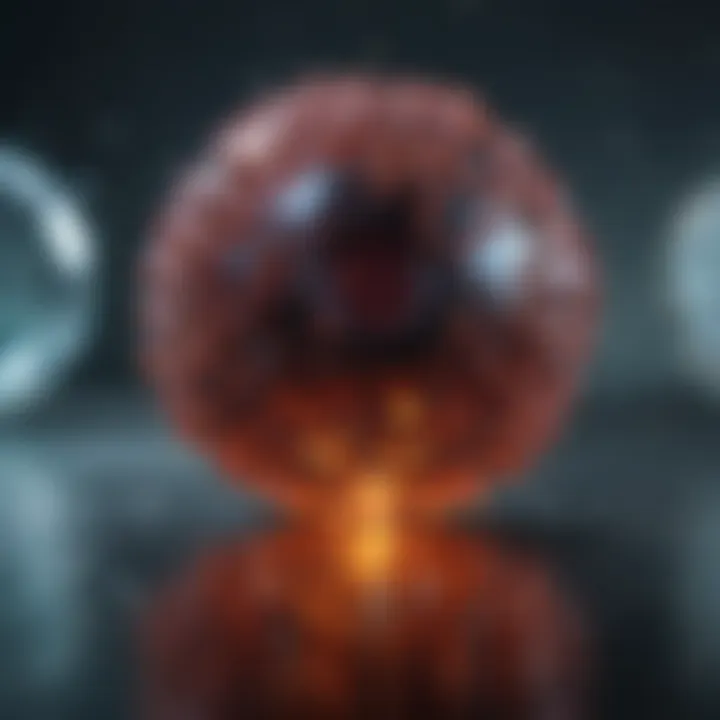
Biomedical Imaging
Biomedical imaging is a field that greatly benefits from SERS nanoparticles. These nanoparticles enable enhanced imaging of biological tissues, distinguishing between healthy and diseased cells with remarkable accuracy. The unique vibrational signatures they produce under specific conditions allow for the identification of molecular changes that may signal early disease progression, such as in cancer.
To illustrate, one might consider a scenario where SERS nanoparticles are applied to visualize tumors in real-time. These particles can be engineered to bind specifically to cancer cell markers, emitting vibrant signals that highlight the tumor area amidst surrounding healthy tissue. Consequently, surgeons can achieve better outcomes by ensuring complete tumor resection. This capability underscores the substantial advantages of SERS-driven imaging techniques over conventional imaging modalities, which often produce blurry or overlapping results.
Biosensing and Diagnostics
SERS nanoparticles are redefining biosensing and diagnostics by providing ultra-sensitive detection of biomolecules, like proteins and DNA, at remarkably low concentrations. This sensitivity is particularly crucial in early-stage disease detection, where the presence of specific biomarkers can indicate the onset of diseases such as Alzheimer's or various forms of cancer.
The diagnostic power of SERS allows clinicians to develop assays that are faster, cheaper, and more accurate. For instance, a SERS-based biosensor can detect specific pathogens in bodily fluids, which is invaluable for rapid diagnosis during outbreaks. The simplicity of the methodology can lead to unexpected breakthroughs, particularly in resource-limited settings where traditional diagnostic approaches might fail.
"The integration of SERS nanoparticles in biosensors marks a pivotal shift in how we approach health diagnostics, enabling a proactive stance in disease management and prevention."
Drug Delivery Systems
The role of SERS nanoparticles in drug delivery systems is emerging as another exciting frontier. These particles can serve as carriers, transporting therapeutic agents directly to target sites within the body, thereby minimizing side effects. They can be functionalized to ensure specificity – essentially creating a delivery system that activates only in the presence of predetermined biological markers. This tailored approach not only enhances the efficacy of the drug but also significantly reduces toxicity to healthy tissues.
Research is ongoing into how these nanoparticles can be combined with existing drug therapies to improve overall treatment outcomes. For example, conjugating chemotherapy drugs with SERS nanoparticles could allow for real-time monitoring of drug dispersal in the body, optimizing dosages without requiring invasive procedures.
In summary, SERS nanoparticles present a wealth of possibilities in biological applications, promising advancements in diagnostic accuracy, imaging precision, and therapeutic effectiveness. As research progresses, it will be fascinating to see how these innovative tools continue to shape the landscape of modern medicine.
Chemical Analysis and Sensing
Chemical analysis and sensing are crucial elements in the study and application of SERS nanoparticles, providing insights into the compositional makeup of various samples. These technologies are pivotal not just for laboratory settings but also for practical applications in fields like environmental monitoring and industrial processes. The finesse with which SERS can detect and quantify specific chemicals highlights its importance.
When considering chemical analysis, one can’t overlook the unique advantages offered by SERS nanoparticles. Firstly, their high sensitivity means that even minute concentrations of analytes can be traced, making it ideal for detecting pollutants or hazardous substances that may be otherwise overlooked. Moreover, the ability to withhold significant spatial resolution adds an extra layer of utility: you’re not just finding an analyte, but you’re becoming aware of its exact localization within a sample.
Some additional factors underpin the significance of chemical analysis and sensing with SERS include:
- Cost-effectiveness: Compared to some other analytical techniques, SERS can reduce the overall costs associated with chemical analysis without compromising on accuracy.
- Wide application spectrum: From pharmaceuticals to food safety, the versatility of SERS nanoparticles can cater to a broad array of industries.
- Real-time analysis: Many SERS setups allow for the monitoring of reactions and changes in real time, a critical element in various fields.
"The ability of SERS nanoparticles to detect and analyze chemical compounds with precision not only paves the way for advances in science but also has far-reaching implications for public health and safety."
Such factors illuminate why SERS plays a fundamental role in chemical analysis and sensing. Following this, let’s dive deeper into how these particles assist in detecting analytes in solutions specifically.
Detecting Analytes in Solution
In the world of chemical analysis, the capacity to detect analytes dissolved in solutions stands as a significant challenge. However, SERS nanoparticles rise to this occasion, enhancing traditional Raman spectroscopy to achieve detection limits that are often unattainable otherwise. The surface enhancement process means that when light interacts with these nanoparticles, the resultant signal is amplified, allowing even minute traces of substances to be detected.
Detecting analytes in solutions using SERS entails several practical considerations:
- Selection of the substrate is crucial, as it influences the enhancement factors significantly.
- Solvent effects can alter the spectral features, hence understanding the matrix is necessary for accurate interpretation.
- Functionalization of nanoparticles can optimize their interaction with specific analytes, leading to increased selectivity and sensitivity.
Ultimately, these elements are synthesized to provide a robust platform for successfully detecting analytes. With the accuracy and sensitivity of SERS, it's no surprise that researchers have harnessed its capability for diverse applications, particularly in environmental contexts.
Applications in Environmental Monitoring
The significance of SERS nanoparticles extends beyond laboratory doors, finding relevance in the realm of environmental monitoring. As pressing issues like pollution and climate change surface, the need for effective monitoring solutions has never been clearer. Here, SERS nanoparticles facilitate real-time, sensitive detection of pollutants, allowing for timely interventions.
The benefits of utilizing SERS in environmental monitoring can be highlighted as:
- Identification of contaminants: SERS can uncover hazardous chemicals such as heavy metals and organic pollutants in water bodies—substances that might otherwise escape detection.
- Monitoring changes over time: The technology’s ability to track how analyte concentrations fluctuate aids in assessing the effectiveness of remediation strategies.
- Portability and ease of use: Compact SERS setups enable on-site analysis, turning a complex lab process into an accessible tool for field studies.
In summary, the application of SERS nanoparticles in chemical analysis, particularly in detecting analytes in solution and environmental monitoring, addresses urgent scientific and practical challenges. Their unparalleled sensitivity and versatility provide myriad opportunities for better understanding and managing both chemical processes and environmental concerns.
Material Science and Engineering
Material science is at the heart of countless innovations and breakthroughs shaping our modern world. SERS nanoparticles serve as a crucial intersection between materials science and engineering, allowing researchers and technologists to manipulate and enhance materials at the nanoscale. By developing new composites and exploring self-assembly techniques, scientists unlock new possibilities that have significant implications across a variety of sectors, from electronics to biomedical applications.
Nanoparticle Composites
Nanoparticle composites present a fascinating avenue within material science. They merge different materials at the nanoscale to produce unique properties that aren't found in bulk materials. When SERS nanoparticles are embedded in composite materials, they can significantly enhance their functionality. For instance, incorporating silver or gold nanoparticles into a polymer matrix can lead to improved optical properties, making them ideal for sensors, coatings, or even in electronics.
The adaptability of these composites allows for fine-tuning the physical properties through adjustments in the nanoparticle size, shape, and concentration. This tunability is essential, particularly in SERS applications where sensitivity and specificity are paramount. When we consider real-world applications, the implications are vast. In the realm of biomedicine, these composites can enable highly sensitive detection of biomarkers in patient samples, aiding in early diagnosis.
- Enhanced Sensitivity: Due to the plasmonic properties of nanoparticles, composites can improve the enhancement factor in SERS, making detection of low-abundance species feasible.
- Versatile Applications: They can be tailored for specific uses, from environmental monitoring tools to advanced medical diagnostics.
- Increased Durability: Nanocomposites often exhibit improved mechanical properties, allowing them to endure challenging conditions.
Self-Assembly Techniques
Incorporating self-assembly techniques is pivotal in material science, particularly for creating organized structures at the nanolevel. These methods harness the natural tendency of nanoparticles to self-organize into ordered patterns without external guidance. This can simplify manufacturing while considerably reducing costs.
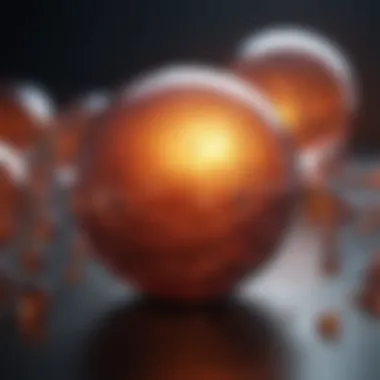
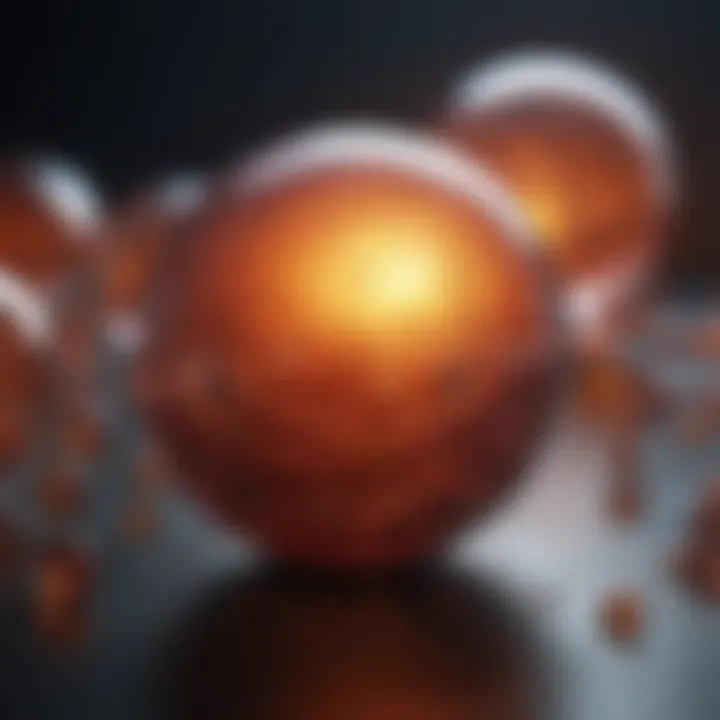
For SERS nanoparticles, self-assembly can improve their spatial arrangement, maximizing the number of enhanced hot spots available for interactions. A well-ordered array of nanoparticles will have higher efficiency compared to a random distribution, leading to superior performance in applications such as sensing.
Key approaches in self-assembly include:
- Layer-by-layer assembly: Alternating layers of positively and negatively charged materials create a robust structure that can be precisely controlled at the nanoscale.
- Hydrophobic interactions: Utilizing the natural affinity of hydrophobic materials can help in organizing nanoparticles into desirable configurations.
- Template-assisted methods: Using templates aids in directing the self-assembly of nanoparticles into specific geometries or networks.
Emerging research suggests that using self-assembly techniques in tandem with SERS nanoparticles could lead to innovative sensing platforms that are both efficient and cost-effective. Ultimately, the combination of nanoparticle composites and self-assembly methods in material science represents a promising frontier. It opens doors not only for advancements in SERS technology but also for pioneering developments in various fields.
Challenges and Limitations of SERS Nanoparticles
While surface-enhanced Raman scattering (SERS) nanoparticles have positioned themselves as transformative agents in numerous scientific domains, acknowledging the challenges and limitations they present is imperative. These difficulties profoundly impact the effectiveness and practical application of SERS technology. Understanding them not only aids researchers in navigating the complexities of the field but also encourages ongoing dialogues aimed at overcoming these hurdles.
Sensitivity Concerns
One of the core challenges associated with SERS nanoparticles is their sensitivity level. Although they are celebrated for enhancing weak Raman signals, various factors can influence their performance. For instance, the concentration of the analyte can significantly alter the detection limits.
- Environmental Influence: Factors like temperature fluctuations, pH, and even ionic strength in solutions can affect the sensitivity. The nanoparticles can become unstable under varying environmental conditions, leading to inaccurate readings.
- Substrate Dependencies: The efficacy of SERS is often contingent upon the substrate to which the nanoparticles are applied. If the substrate is not handled meticulously or cannot sustain the nanoparticles optimally, the spectral enhancement may diminish, undermining the testing process.
Achieving a balance in sensitivity across diverse applications poses substantial challenges. It's like trying to hit a moving target while blindfolded; the finer the sensitivity, the higher the risk of false negatives and positives, which can introduce ambiguity in crucial studies across fields such as pharmaceuticals and environmental science.
Reproducibility Issues
Another significant limitation lies in the reproducibility of results obtained from SERS nanoparticles. Researchers often find that different batches of nanoparticles yield variable outcomes during experiments, which raises eyebrows in scientific validity.
- Fabrication Variability: The synthesis process, whether top-down or bottom-up, can result in nanoparticles that differ in size, shape, and morphology. These discrepancies can lead to inconsistencies in enhancement factors, causing experiments to be less reliable.
- Measurement Parameters: Variability can also arise during measurements. Different setups and operators can introduce unique biases, resulting in variability in spectral data that might mislead interpretations.
- Standardization Gap: The lack of standardized protocols for evaluating SERS nanoparticles means results can vary across laboratories. Each team might bring different practices and equipment into play, leading to a disparate quality of results.
Reproducibility is akin to planting a garden — without the right conditions and uniformity, what flourishes in one setting might barely survive in another. This variability can vex scientists, preventing them from establishing robust benchmarks for practical applications.
"Addressing sensitivity and reproducibility issues is paramount to unlocking the full potential of SERS nanoparticles in diverse scientific applications."
Future Directions in SERS Research
The exploration of future directions in SERS research is crucial for harnessing its full potential across scientific disciplines. This area not only reflects the cutting-edge innovations but also highlights how quickly technology can adapt to meet the challenges posed by real-world applications. As researchers continue to delve into the intricate world of SERS nanoparticles, they must consider the ethical implications, scalability, and integration into existing scientific frameworks.
Emerging Technologies and Applications
The dawn of novel techniques has paved the way for exciting developments in SERS. One major trajectory lies within the integration of artificial intelligence (AI) and machine learning with SERS technology. By applying these advanced methods, researchers can optimize the synthesis of nanoparticles and improve the predictiveness of spectral outputs. This could lead to a more customized approach to biosensing, enhancing sensitivity and specificity in detecting biomolecules.
Moreover, advances in nanofabrication techniques like 3D printing hold promise for creating intricate SERS substrates. These substrates can enhance detection processes by providing a rich array of active sites for molecules to bind. When coupled with nanostructures that exhibit unique optical properties, the sensitivity can reach unprecedented levels.
In addition, multi-modal imaging techniques combining SERS with other imaging modalities could provide a more holistic view of biological processes. For instance, integrating SERS with fluorescence imaging enables simultaneous monitoring of different biomolecules, leading to greater insights into cellular dynamics.
Here are some points regarding emerging applications:
- Medical Diagnostics: SERS can potentially revolutionize point-of-care testing by allowing rapid detection of pathogens and biomarkers, improving patient outcomes.
- Environmental Monitoring: As climate change concerns grow, SERS applications in detecting pollutants and toxins in water and air quality testing are becoming increasingly relevant.
- Food Safety: Nanoparticles can monitor food products for contaminants, ensuring safety and compliance with health regulations.
"Emerging technologies in SERS are not just about improvements in detection. They're transforming how we understand complex systems across various fields."
Integration with Other Analytical Techniques
To further expand the applications of SERS, integrating it with other analytical techniques is paramount. The combination of SERS with methods such as mass spectrometry or high-performance liquid chromatography (HPLC) can result in powerful analytical toolsets. These integrations can enhance sensitivity and specificity while allowing for the simultaneous analysis of multiple compounds.
For example, in the realm of drug delivery tracking, merging SERS with mass spectrometry could provide rich qualitative and quantitative data about the drug's lifecycle within biological systems. This holistic approach helps inform not just drug efficacy but also safety profiles.
Furthermore, employing SERS in conjunction with imaging techniques like MRI or PET could revolutionize cancer diagnostics. It enables visualization and quantification of specific biomarkers associated with tumors, making it possible to track disease progression in real-time.
In summary, the synergies created by integrating SERS with other analytical tools are not just adding layers of complexity; instead, they open doors to previously uncharted territories in scientific research and practical applications. As we look to the future of SERS research, the focus on these integrations will only grow stronger, fostering an environment where groundbreaking discoveries can flourish.
Closure
Understanding the role of SERS nanoparticles and their diverse applications is like piecing together a complex puzzle. Each aspect discussed in this article shines a light on how SERS technology has been integrated into different scientific disciplines, marking its relevance and transformative potential for future research. This conclusion serves to tie together the threads of our exploration, emphasizing key elements that underscore the importance of these nanoparticles in modern science.
Summary of Key Findings
In the body of this article, we've navigated through several critical points:
- Mechanisms of Surface Enhancement: The electromagnetic and chemical mechanisms that lend to the impressive sensitivity of SERS were elaborated, showcasing the influence of nanoparticle morphology on enhancement factors.
- Synthesis of SERS Nanoparticles: Various approaches like top-down and bottom-up methods reveal opportunities for creating tailored nanoparticles that could meet specific research requirements. Functionalization techniques enhance their application range.
- Characterization Techniques: Reliable techniques for morphological analysis and spectroscopy were highlighted. These methods are pivotal in ensuring the stability and reproducibility of nanoparticles, vital for any scientific inquiry.
- Biological and Chemical Applications: The discussions included how SERS nanoparticles serve as tools in biomedical imaging, biosensing, and even drug delivery, indicating their indispensable role in advancing healthcare technology. Additionally, their utility in monitoring environmental conditions illustrates the versatility of these particles across domains.
- Challenges and Future Directions: Sensitivity and reproducibility challenges were candidly addressed. Looking ahead, emerging technologies could facilitate overcoming these drawbacks while integrating SERS nanoparticles with other analytical techniques offers exciting pathways for exploration.
Final Remarks on the Impact of SERS
The impact of SERS nanoparticles is profound, shaping the landscape of scientific research across fields. These particles are not merely a passing trend; they are instrumental in catalyzing advancements that could redefine our understanding of molecular interactions.
"SERS technology stands at the crossroads of analytical technique and application; it paves the way for future studies that demand both precision and versatility."
While the road ahead may contain hurdles related to sensitivity and reproducibility, the potential benefits of these nanoparticles promise a brighter future for researchers and industries alike. Their ability to facilitate high-resolution analysis and real-time detection of substances could lead to groundbreaking discoveries, especially in diagnostics, environmental science, and material development.
In essence, the multifaceted applications of SERS nanoparticles underline a pivotal turning point in scientific innovation. As we move forth in the ever-evolving world of nanotechnology, keeping an eye on the developments in SERS research will surely yield rich insights that can drive both theoretical and practical advancements.