Energy Production from Biomass: A Comprehensive Review
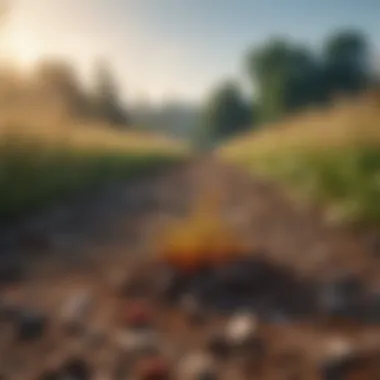
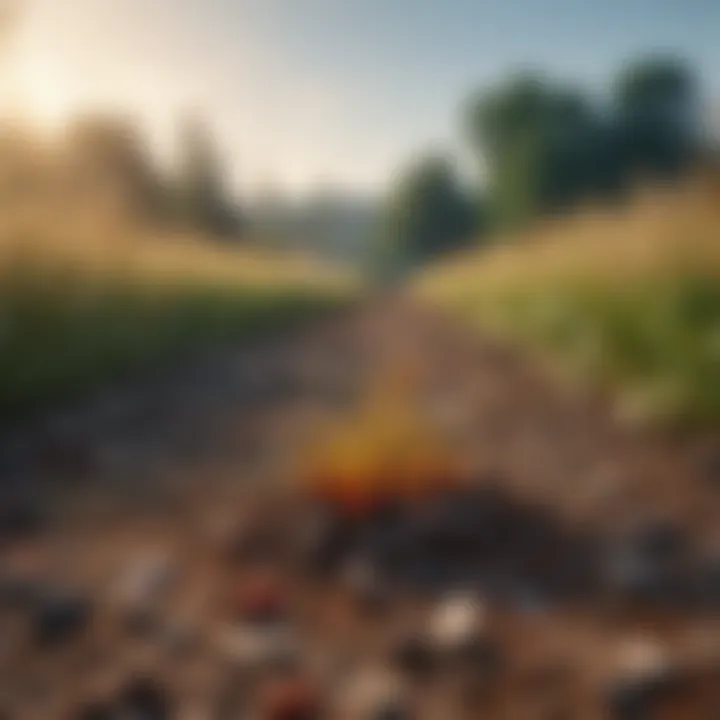
Research Overview
Energy production from biomass presents a promising avenue in the broader scope of renewable energy sources. As global energy demands continue to rise while the impacts of fossil fuel consumption become increasingly apparent, biomass offers a practical solution to both fuel needs and waste management challenges.
Summary of Key Findings
Biomass can be sourced from various materials, including agricultural residue, forestry byproducts, and organic municipal waste. These sources can be converted into energy through several technologies, each with unique advantages and drawbacks. Notably, the application of these technologies can yield significant ecological benefits if managed correctly.
"Harnessing biomass not only helps meet energy needs but also enhances waste management strategies, creating a greener future."
The production of energy from biomass can occur through several processes such as combustion, gasification, and anaerobic digestion. Each of these methods has its specific mechanisms and is suitable for different types of biomass feedstock.
Background and Context
Historically, biomass has been utilized as a primary energy source for millennia. However, the recent emphasis on sustainability and renewable energy has reignited interest in its potential. The quest for cleaner energy alternatives, combined with advancements in conversion technologies, places biomass at a crucial intersection of energy, environment, and economy. Understanding the role of biomass can help shape policies and practices that support a sustainable energy future.
Methodology
Research into the energy production from biomass is multifaceted, incorporating both empirical studies and theoretical analyses. This section discusses the framework and approach taken in exploring this important topic.
Experimental Design
The exploration of energy production systems generally relies on a combination of laboratory experiments and real-world applications. These varied approaches ensure that findings are not only theoretically grounded but also practically applicable in diverse settings.
Data Collection Techniques
Data on biomass conversion is typically collected via multiple avenues, including:
- Field Trials: Real-world implementations provide data on efficiency, emissions, and outputs.
- Laboratory Analysis: Controlled experiments measure the energy yield and byproduct generation.
- Surveys and Case Studies: Public and industrial feedback helps assess the socio-economic impact of biomass energy systems.
Through a blend of these methodologies, the potential of biomass as a sustainable energy source can be thoroughly evaluated, allowing for informed decisions in the transition towards renewable energy futures.
Foreword to Biomass Energy Production
Biomass energy production represents a crucial area of renewable energy research and implementation. This section delves into the foundation of biomass as an energy source, underlining its significance amid the global shift towards sustainable energy practices. In essence, biomass energy is derived from organic materials, which are plentiful and diverse.
The production of energy from biomass not only addresses energy demands but also contributes to effective waste management, reducing landfill burdens and promoting a circular economy. Additionally, as nations grapple with climate change issues, biomass emerges as a renewable alternative that can help decrease greenhouse gas emissions when managed sustainably. It creates new markets and job opportunities while fostering energy security on both local and national levels.
Definition of Biomass
Biomass refers to biological materials, particularly plant and animal matter, which can be utilized as fuel for energy production. This includes a variety of sources, such as agricultural residues, forestry by-products, and even dedicated energy crops. By converting these materials into usable energy, we can harness the energy stored in their chemical bonds. Biomass is not only renewabie but also can be sustainably sourced when harvested correctly, making it a key player in current and future energy strategies.
Historical Context
The history of biomass energy use traces back thousands of years. Early civilizations used wood and animal waste for heating and cooking. With industrialization, the reliance on fossil fuels increased, sidelining biomass to some degree. However, as the adverse effects of fossil fuel usage have became clearer, interest in biomass has resurfaced.
In the latter half of the 20th century, research and technology advancements began to focus on biomass as an energy solution. Policies aimed at energy independence and environmental protection have further fueled this interest. Today, numerous countries are investing in biomass technologies and infrastructure to improve energy production and sustainability.
"Biomass energy presents a viable solution as we transition towards cleaner energy sources, underlining the importance of sustainable practices in energy production."
The integration of biomass energy production into the global energy landscape showcases both its historical significance and future potential. Understanding the nuances of biomass energy is vital for policymakers, researchers, and the general public as they navigate the energy challenges of the modern world.
Types of Biomass Resources
Understanding the types of biomass resources is crucial to grasp the various potential and mechanisms behind biomass energy production. Each type serves a unique purpose, contributing to the overall sustainability goals by turning waste into energy. Utilizing biomass resources effectively can reduce waste disposal issues while providing alternative energy solutions. The significant categories include agricultural residues, forestry by-products, animal manure, and dedicated energy crops. Each resource possesses specific characteristics that influence their conversion technologies and end products.
Agricultural Residues
Agricultural residues are the leftover materials from crop production. These materials include straw, corn stover, and plant trimmings. They represent a considerable opportunity for biomass energy, as they are abundantly available in farming regions. Turning agricultural residues into energy helps farmers not only manage waste but also gain additional revenue streams. By integrating biomass energy production in traditional agriculture, farmers can create a more sustainable agricultural system, reducing dependency on fossil fuels.
Some benefits include:
- Waste Reduction: Effective use of residues helps minimize waste in agricultural activities.
- Economic Incentives: Farmers can sell these materials to biomass energy facilities, improving their profit margins.
- Soil Improvement: Properly managed residues can also enrich soil quality when returned to fields after processing.
Forestry By-products
Forestry by-products comprise waste materials generated from timber and wood processing, such as sawdust, wood chips, and bark. These by-products often become waste if not utilized efficiently. However, they are resourceful in biomass energy production, providing a renewable source of energy in regions with considerable forest management activities. Utilizing forestry by-products can significantly decrease landfill pressures and enhance sustainable forestry practices.
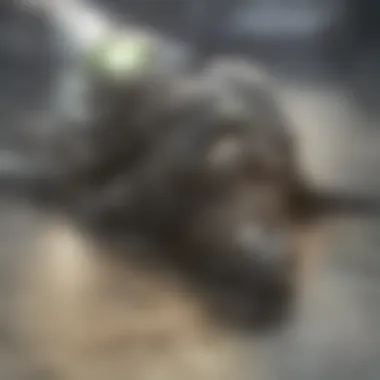
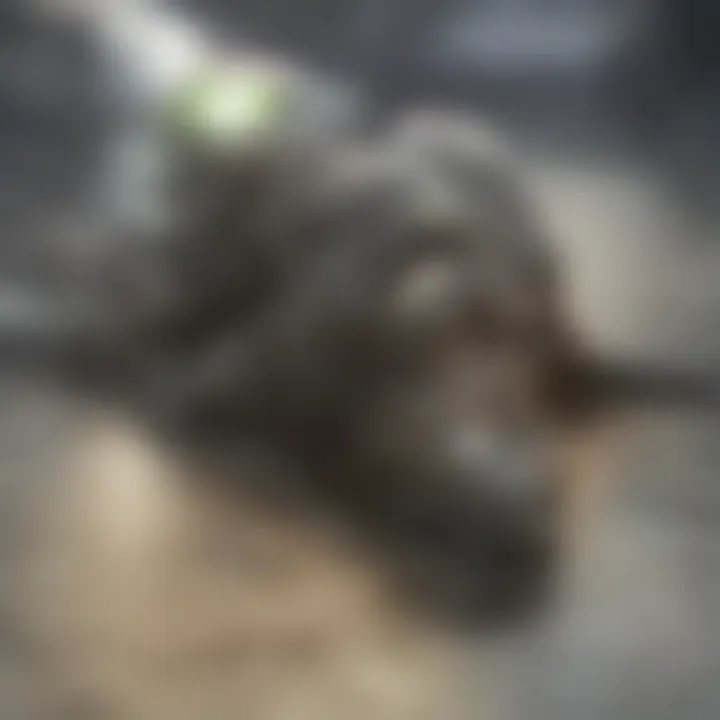
Key points include:
- Resource Efficiency: Enhancing the energy extraction from wood materials ensures a comprehensive utilization of harvested trees.
- Carbon Sequestration: Utilizing wood waste contributes to carbon neutrality as it reduces overall emissions from burning conventional fuels.
- Economic Utilization: Forestry operations can diversify income sources by venturing into biomass energy production.
Animal Manure
Animal manure serves as another crucial biomass resource. Livestock operations generate vast amounts of manure, which can be converted into energy through various conversion technologies. By turning manure into biogas or biofertilizer, farms can not only manage waste but also create a circular economic model, providing them energy and enhancing soil productivity.
Benefits of using animal manure include:
- Energy Generation: Potential to produce biogas, which can be utilized for electricity and heat.
- Nutrient Recycling: Converts waste into fertile bioproducts that can be used to enrich soils.
- Greenhouse Gas Reduction: Efficient management of manure reduces methane emissions associated with decomposition.
Dedicated Energy Crops
Dedicated energy crops are specifically cultivated with the intent of biomass energy production. These crops are chosen for their high yield potential and adaptability to a variety of environmental conditions. Crops like switchgrass, miscanthus, and giant reed can be grown without displacing food production, making them a strategic addition to energy portfolios.
Some essential considerations include:
- High Yields: These crops often produce more biomass per acre compared to traditional crops, maximizing energy output.
- Sustainability: Growing dedicated crops can provide a steady supply of biomass while supporting soil health.
- Diverse Applications: Energy crops can be converted into various energy products, including ethanol and biofuels, expanding their utility.
Through understanding these various biomass resources, stakeholders can make informed decisions that advance both energy and environmental goals. The diverse options available showcase the adaptability and potential of biomass in the broader context of renewable energy.
Conversion Technologies for Biomass Energy
Understanding the various conversion technologies for biomass energy is crucial in realizing the potential of biomass as a renewable energy source. These technologies determine how effectively biomass can be transformed into usable energy forms. Each method offers specific benefits and challenges that dictate its applicability and efficiency.
Thermochemical Conversion
Thermochemical conversion refers to the process that uses heat to convert biomass into energy. This process uses high temperatures to break down biomass materials. The two primary methods under thermochemical conversion are gasification and pyrolysis.
Gasification
Gasification is a process that converts organic or fossil-based materials into carbon monoxide, hydrogen, and carbon dioxide. This method involves heating biomass in a low-oxygen environment. One key characteristic of gasification is its ability to create syngas, a fuel that can be further processed into synthetic natural gas or liquid fuels. This makes gasification a beneficial choice in producing cleaner energy.
Its unique feature is that it can utilize various feedstock, from agricultural waste to forestry residues. However, disadvantages include the need for complex technology and equipment. Additionally, the process can produce harmful by-products if not managed correctly.
Pyrolysis
Pyrolysis is another thermochemical conversion method. It involves heating biomass in the absence of oxygen to produce bio-oil, syngas, and char. This method is popular due to its versatility in generating multiple products. A distinctive aspect of pyrolysis is its ability to convert biomass into bio-oil, which can be upgraded to renewable fuels.
The unique feature of pyrolysis is its relatively lower temperature requirement compared to gasification. Its advantages include high energy yield and the option to produce solids like charcoal. However, challenges lie in the quality of the bio-oil, which can vary based on the feedstock and conditions.
Biochemical Conversion
Biochemical conversion relies on biological processes to convert biomass into energy, mainly through the action of microorganisms. Anaerobic digestion and fermentation are the two main types in this category.
Anaerobic Digestion
Anaerobic digestion is a natural process in which microorganisms break down organic materials in the absence of oxygen. The importance of this technique lies in its ability to convert organic waste into biogas and digestate. Biogas is a valuable renewable energy source that can be used for heating or electricity generation.
The key characteristic of anaerobic digestion is its efficiency in waste management, taking advantage of materials that would otherwise enter landfills. Its unique feature is the simultaneous production of energy and nutrient-rich fertilizer, which supports sustainability practices. On the downside, the process requires careful monitoring and can be slow.
Fermentation
Fermentation is a biochemical process that converts sugars into alcohol or organic acids using microorganisms. This process is primarily used to produce ethanol, a significant alternative fuel. Fermentation is crucial for its contributions to reducing reliance on fossil fuels.
Its key characteristic is the requirement for carbohydrate-rich feedstock such as corn or sugarcane, which can be abundant. The unique feature of fermentation is its adaptability across different biomass types. However, drawbacks include competition with food resources and the cost of feedstock.
Physical Processes
Physical processes involve mechanical techniques that enhance the density and handling properties of biomass before energy conversion. The two main processes are compaction and pelletization.
Compaction
Compaction is the process of compressing biomass materials into denser forms. This technique is especially vital for transportation and storage, addressing challenges related to the volume of raw biomass. The main advantage of compaction lies in its capacity to reduce shipping costs through lower volume.
The unique feature of this process is that it can create briquettes from various feedstock. Nevertheless, the energy required for compaction can undermine its overall efficiency.
Pelletization
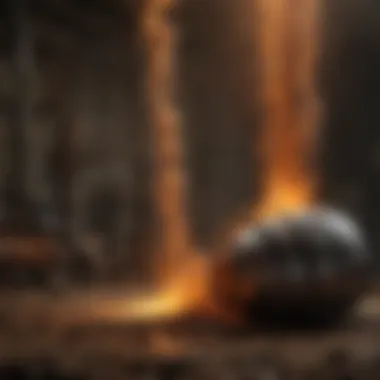
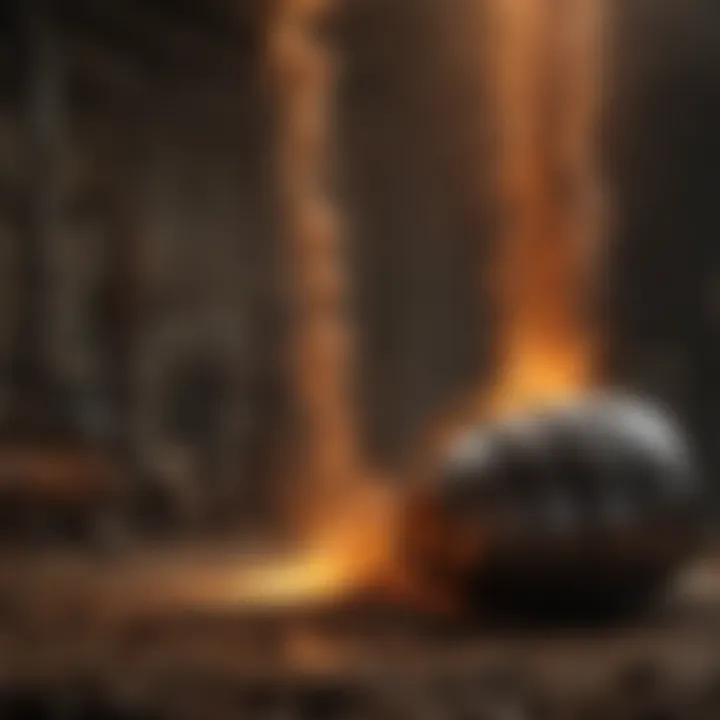
Pelletization takes the compaction process further. It involves creating small pellets from biomass through mechanical pressure and high temperatures. This method enhances the biomass's energy density and enhances uniformity, making it easier for combustion.
The key characteristic of pelletization is its high energy output compared to loose biomass. A unique feature is its capability to produce high-quality fuels for industrial and residential heating systems. However, the initial investment for pelletizing equipment can be significant, somewhat limiting its accessibility.
Environmental Impact of Biomass Energy
Understanding the environmental impact of biomass energy is essential for evaluating its role as a sustainable energy source. This section explores various dimensions, highlighting the benefits and considerations that come with using biomass for energy production. It is vital to assess not only the potential advantages but also the ecological footprints left by such practices, ensuring that biomass is a genuinely renewable alternative.
Carbon Footprint Analysis
The carbon footprint analysis of biomass energy production examines the net greenhouse gas emissions. Biomass is often considered carbon-neutral because the carbon dioxide released during combustion is roughly equivalent to the amount absorbed by plants during their growth. However, this perspective requires a deeper understanding.
- Emissions during Growth and Harvest: The cultivation, harvesting, and processing of biomass often involve emissions from fertilizers, machinery, and transportation.
- End-use Emissions: While the combustion of biomass emits carbon dioxide, other gases such as methane and nitrous oxide may also be released, contributing to the overall greenhouse gases in the atmosphere.
- Life Cycle Assessment: Conducting a comprehensive life cycle assessment is important. This method considers all stages, from production to disposal, ensuring emissions are minimized at every point. Understanding the carbon fluxes through each stage allows for better management and mitigation of potential impacts.
Land Use Changes
Land use changes related to biomass energy involve significant implications for ecosystems and agriculture. As demand for biomass increases, it can lead to:
- Conversion of Natural Habitats: Deforestation or conversion of grasslands for energy crop production can threaten biodiversity. Habitats may be destroyed, affecting local wildlife.
- Agricultural Land Competition: Utilizing land for biomass can compete with food production. This shift can lead to increased food prices and food security concerns, particularly in developing regions.
- Soil Health and Land Degradation: Intense cultivation of biomass crops can lead to soil degradation, erosion, and loss of fertility over time. Sustainable practices are necessary to maintain soil integrity and prevent long-term damage.
Proper planning and strategic land use policies can mitigate these changes. Informed decisions will help balance energy production needs with conservation efforts.
Biodiversity Implications
The use of biomass energy presents both challenges and opportunities for biodiversity. The implications are critical and require careful assessment:
- Ecosystem Services: Biomass production can disrupt the natural ecosystem balance. As land is repurposed, the services provided by biodiversity—such as pollination, water purification, and carbon sequestration—may be adversely affected.
- Monoculture Practices: Large-scale biomass production often promotes monoculture farming, which threatens plant and animal species. This reduces genetic diversity, making ecosystems more vulnerable to diseases and climate change.
- Restoration Potential: Conversely, when managed sustainably, biomass production can foster habitat restoration. Certain bioenergy projects can encourage the conservation of degraded lands, promoting native vegetation and biodiversity recovery.
"The challenge lies in striking a balance between energy needs and preserving the ecological integrity of our planet."
The future of biomass energy will largely depend on its integration within broader biodiversity conservation strategies. Effective policies should prioritize both renewable energy production and the safeguarding of natural resources.
Economic Considerations in Biomass Energy
Understanding the economic considerations in biomass energy is crucial for evaluating its viability as a renewable energy source. This section will explore various aspects that impact the production and utilization of biomass energy, shedding light on costs, market dynamics, and relevant government interventions. These considerations not only define the economic landscape but also influence the wider adoption of biomass as an alternative energy solution.
Cost of Biomass Production
The cost of biomass production can fluctuate depending on several factors, including the type of biomass resource, production methods, and location. To get an accurate picture, we must take into account both direct and indirect costs involved in biomass energy production.
- Raw Material Costs: The collection and transportation of biomass feedstock play a significant role in overall production costs. Sources might be agricultural residues, forestry by-products, or dedicated energy crops. Each has its associated costs based on accessibility and availability.
- Processing Costs: Conversion processes, whether thermochemical or biochemical, require investment in technology and infrastructure. Facilities for gasification, pyrolysis, and anaerobic digestion have their operational expenses that must be carefully accounted for.
- Economies of Scale: Larger operations may benefit from economies of scale. This allows for reduced average costs per unit as production increases, making it more viable in competitive energy markets.
- Market Fluctuations: Prices of biomass feedstock can be volatile due to changing agricultural conditions, energy demand, and competition from other uses for the raw materials.
Market Demand and Supply Dynamics
Market demand for biomass energy is influenced by a variety of trends within the energy sector. Understanding these dynamics is essential for stakeholders involved in biomass energy production.
- Growing Energy Needs: As global energy demands increase, the search for renewable alternatives becomes critical. Biomass energy offers a potential solution, thereby increasing its market demand.
- Environmental Considerations: Many companies are shifting towards sustainable practices to reduce their carbon footprints. This boosts the demand for biomass as firms look to integrate cleaner energies into their operations.
- Compatibility with Existing Infrastructure: Biomass can often be integrated into existing energy systems including co-firing in coal plants. This compatibility fosters greater acceptance and demand in the market.
- Supply Chain Challenges: Fluctuations in the supply of biomass due to harvests, land use changes, and transportation issues can affect market stability. Companies must remain agile to adapt to these changes.
Government Policies and Incentives
Government policies play a pivotal role in shaping the biomass energy landscape. Incentives and regulations can facilitate or hinder the growth of biomass energy production.
- Subsidies and Financial Incentives: Many governments provide subsidies for biomass production to stimulate growth. This can help offset the high initial costs associated with biomass energy setup.
- Regulatory Frameworks: Regulations governing sustainability and emissions can significantly impact the profitability of biomass projects. Clear and supportive policies encourage investment and help standardize production practices.
- Research and Development Funding: Governments often allocate resources towards R&D in biomass technologies. This funding can lead to better conversion technologies and more economical production processes.
- Renewable Energy Targets: By setting renewable energy targets, governments create a favorable market environment for biomass energy. Compliance with these targets can be crucial for countries aiming to transition to greener energy.
"Investing in biomass energy is not simply a financial decision; it is an opportunity to align with global sustainability goals and meet future energy demands."
Challenges in Biomass Energy Production
The journey toward effective biomass energy production is not without its challenges. Understanding these obstacles is crucial for optimizing the use of biomass as a renewable energy source. Factors like technological barriers, regulatory concerns, and public perception directly impact the feasibility and sustainability of biomass energy projects. Identifying these aspects offers insights into the current state of biomass energy and its potential future.
Technological Barriers
Technological barriers pose significant challenges in the biomass energy sector. The conversion of biomass into energy relies on various technologies, each with its own complexities. For instance, thermochemical processes like gasification and pyrolysis require advanced equipment and control systems to operate efficiently. The need for high-temperature and high-pressure operations can make these technologies expensive and impractical for smaller operations.
Moreover, the efficiency of conversion processes is often inadequate. Low conversion rates lead to greater biomass consumption for lower energy output, which is economically unviable. Research and development in this field are critical to improve existing technologies and develop new methods that enhance energy output while reducing costs.
Regulatory Concerns
Regulatory concerns play a vital role in the biomass energy landscape. Governments establish regulations that can either promote or hinder biomass production. Inconsistent regulations across different regions can create uncertainty for investors and developers. Lack of clear guidelines often results in delays in project approvals and increases compliance costs.
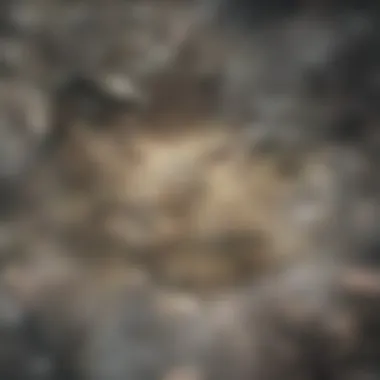
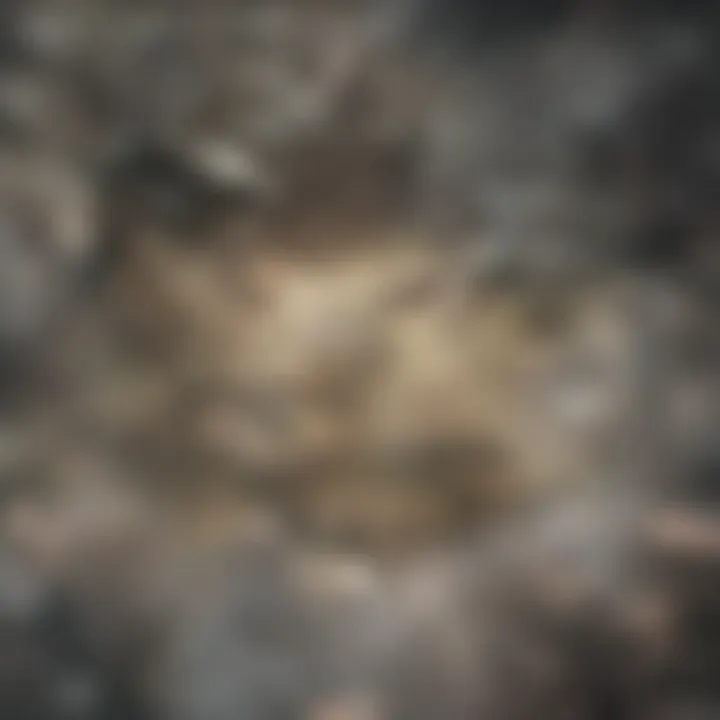
Additionally, regulations pertaining to land use and environmental impact can restrict the availability of biomass resources. Striking a balance between sustainable biomass production and regulatory frameworks is essential. Policies should facilitate growth while ensuring minimal environmental disruption.
Public Perception and Acceptance
Public perception and acceptance are critical to the acceptance of biomass energy. Negative perceptions can stem from misinformation about sustainability and environmental impacts. Some stakeholders may oppose biomass projects due to concerns over land use, deforestation, and emissions, despite biomass being a renewable resource.
Effective communication and education are key. It is essential to provide clear information on the benefits and the responsible practices involved in biomass production. Public engagement can help build trust and understanding, leading to greater acceptance of biomass energy initiatives.
Stakeholder engagement and transparent communication can mitigate public concerns and enhance project viability.
Case Studies in Biomass Energy Applications
Case studies in biomass energy applications are critical to understanding how this energy source can be harnessed effectively. They showcase real-world examples of successful biomass utilization, helping to highlight the potential benefits and challenges associated with implementing biomass energy solutions in various settings. By examining these cases, we can draw valuable lessons and insights that can guide future endeavors in the field.
The exploration of these case studies also provides a platform for evaluating different biomass conversion technologies and their operational viability. Furthermore, they allow stakeholders, including policymakers, researchers, and investors, to assess the economic and environmental implications of biomass energy projects. The richness of these examples often reveals how localized conditions—such as resource availability, technological readiness, and community engagement—can influence outcomes.
Successful Implementation in Europe
Europe has emerged as a leader in biomass energy applications, implementing effective strategies to integrate biomass into their energy landscape. Several countries have adopted policies that support the growth of biomass as a renewable resource. For instance, countries like Sweden and Finland have successfully incorporated biomass into their heating systems, moving away from fossil fuels.
One noteworthy example is the combined heat and power (CHP) plant in Värmland, Sweden. This facility utilizes wood chips sourced from local forestry operations. By converting these wood chips into energy, the plant not only provides heat and electricity to nearby communities but also supports sustainable forest management practices. This success story illustrates the potential of biomass energy to deliver environmental and economic benefits.
Moreover, Germany has developed significant infrastructure for biomass utilization. The country's energy policy promotes the use of biogas, generated from agricultural residues and manure. Many farms across Germany have implemented anaerobic digestion systems to convert their waste into valuable energy, contributing to the nation's renewable energy objectives. These initiatives have formed a basis for further research and innovation in biomass applications.
Innovative Projects in North America
North America has also seen notable innovative projects in the biomass sector, particularly in the United States and Canada. Various initiatives spotlight how diverse biomass sources can be innovatively used for sustainable energy production.
One prominent example is the Salinas Valley sustainable agriculture project in California. This initiative focuses on converting agricultural waste, such as leftover crops, into biofuel. The project not only addresses waste management issues in the agricultural sector but also aims to generate cleaner energy alternatives for local communities. It demonstrates the potential for circular economy principles within the biomass sector.
In Canada, the Wood Pellet Association has facilitated significant advances in utilizing wood residues for energy. Projects in British Columbia showcase the production of high-quality wood pellets from sawmill waste. These pellets are then utilized in residential and commercial heating. This approach not only reduces waste but also provides a new revenue stream for local wood product manufacturers.
Both Europe and North America underscore the viability of biomass energy through these case studies. They highlight tailored solutions to energy challenges, with a focus on sustainability and community involvement. As the demand for renewable energy grows, understanding these case studies becomes pivotal to mapping the future of biomass energy production.
Future of Biomass Energy Production
The future of biomass energy production is pivotal as the world seeks sustainable solutions to meet growing energy demands. Biomass energy offers a renewable alternative to fossil fuels, with a dual advantage of waste management. The importance of advancing biomass technology cannot be overstated, as it holds the potential to reduce greenhouse gas emissions and contribute positively to the global energy mix. By examining emerging technologies and evolving policy frameworks, stakeholders can create an effective roadmap for the development of this green energy source.
Emerging Technologies
Recent advancements in biomass energy technologies indicate a promising trend. These innovations focus on maximizing efficiency and minimizing environmental impact. Some key emerging technologies include:
- Advanced Gasification: This process converts biomass into synthetic gas through high-temperature treatment, leading to cleaner energy output.
- Anaerobic Digestion Innovation: Enhanced methods in biogas production are now capable of increasing yields and improving digestate quality.
- Biomass Hydrothermal Liquefaction: This technology converts wet biomass into bio-oil at lower costs, making it more feasible for large-scale deployment.
- Microbial Fuel Cells: This method uses bacteria to convert organic material directly into electricity, showing great potential for future applications.
These technologies will help to optimize biomass conversion processes. This can also make biomass energy more competitive with other renewables, such as solar and wind.
Policy Trends and Future Directions
Policy frameworks are crucial in shaping the future of biomass energy production. Governments worldwide are recognizing the importance of renewable energy and shifting their focus towards supportive policies that encourage biomass utilization. Several trends are emerging:
- Incentives for Research and Development: Increased funding for innovative biomass solutions fosters competition and drives advancement.
- Regulatory Support: Streamlined permitting and regulatory processes enhance project feasibility, enabling quicker market entry for new technologies.
- Sustainability Standards: Developing clear criteria for sustainable biomass sourcing ensures that environmental integrity is maintained.
- Global Cooperation: Collaboration between nations can lead to shared knowledge and technology transfer, amplifying the growth of biomass energy globally.
"The unique potential of biomass as a versatile energy source signifies a crucial element in the transition to sustainable energy systems."
The integration of these policies will not only propel biomass energy forward but also enhance its role in achieving climate goals. As innovations in technology advance, adapting policies will be essential to leverage the full potential of biomass production, making it a cornerstone for future energy strategies.
Epilogue
In exploring the topic of biomass energy production, this conclusion serves as a synthesis of the insights gained throughout the article. The multifaceted nature of energy from biomass emphasizes its significance in today's renewable energy landscape. Biomass is not merely an alternative source of energy but a potential cornerstone in achieving energy sustainability while addressing waste management issues.
A key point is the variety of biomass sources available. From agricultural residues to dedicated energy crops, each source presents unique advantages and challenges. Understanding these types and their implications is crucial for effective energy planning and implementation. Environmental considerations such as carbon footprint and biodiversity must also be integral to discussions around biomass energy. Economic factors cannot be ignored either; the costs associated with biomass production and market dynamics significantly affect its viability as an energy source.
Additionally, the challenges faced in biomass energy production are substantial. Technological barriers, regulatory concerns, and public perception issues can hinder progress and implementation. Recognizing these challenges is vital for stakeholders, allowing for informed decision-making and strategic planning.
"The potential of biomass as a renewable energy source lies not only in its availability but also in the strategic approach to its utilization."
Overall, the importance of biomass energy production extends beyond mere energy generation. It encompasses environmental stewardship and economic opportunities, paving the way for a more sustainable future. By reviewing the key points presented, one can understand the nuanced role biomass plays in the broader energy transition.
Summary of Key Points
- Diverse Biomass Sources: Biomass comes from various sources including agricultural residues, forestry by-products, and dedicated energy crops. Each source has specific characteristics that determine its utility.
- Environmental Impact: Addressing the carbon footprint and biodiversity implications of biomass energy use is essential. These factors influence public acceptance and regulatory support.
- Economic Considerations: The cost of production and market dynamics significantly dictate the feasibility of biomass energy projects. Supportive government policies can also enhance economic attractiveness.
- Challenges in Implementation: Identifying and addressing technological barriers, regulatory hurdles, and societal acceptance is critical for successfully harnessing biomass energy.
Final Thoughts on Biomass Energy
As the world shifts towards renewable energy, biomass stands as a crucial player. It offers a sustainable solution to energy demands while mitigating the waste crisis. The roadmap to expanding biomass energy production involves continuous innovation in conversion technologies and commitment to environmental and economic metrics.