Exploring Brain Activity Sensors: Innovations and Impact
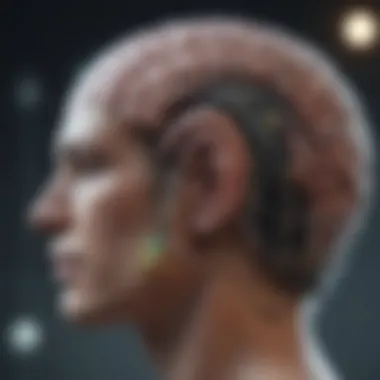
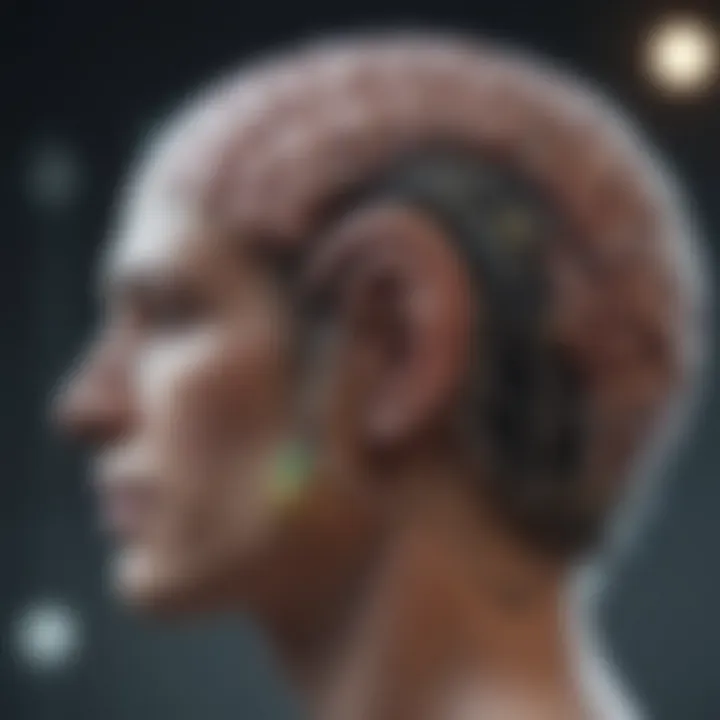
Intro
The emergence of brain activity sensors has sparked an intriguing dialogue within both neuroscience and technology. As we voyage into their depths, we find ourselves contemplating not only their capabilities but also their profound implications for our understanding of the human mind. These sensors have transformed how we approach mental health treatment, human-machine interaction, and cognitive research, revealing intricate patterns of thought and behavior that were once shrouded in mystery.
Understanding this technology isn't just for academics; it has a practical application in everyday life. Consider, for instance, the potential for these sensors to monitor stress or anxiety levels in real-time. Such insights could prompt timely interventions, thereby significantly improving mental well-being. However, jumping into this arena isn't without its ethical quagmires, which we must navigate carefully as we innovate.
Just as every story has its roots, the journey into brain activity sensing begins with a thorough examination of its foundational elements. Context becomes pivotal to grasp the sweeping changes these technologies herald, and that’s what we aim to unpack."
Research Overview
Summary of Key Findings
Recent explorations into brain activity sensors have revealed several noteworthy findings:
- Technological advancements have made sensors more accessible and accurate.
- Applications range from clinical settings for mental health treatment to enhancements in user interfaces, enabling seamless interaction between humans and machines.
- Ethical dilemmas often arise, particularly concerning privacy and consent when dealing with sensitive personal data.
- Interdisciplinary collaboration between fields like neuroscience, engineering, and ethics is key to navigating these innovations.
Background and Context
To fully appreciate the implications of brain activity sensors, it’s crucial to understand their background. Historically, the field has evolved alongside developments in neuroscience and computing.
The idea of tapping into brain activity isn’t brand-new, yet technological barriers often limited it. However, with advancements in materials science and computing power, we can now measure neural signals with remarkable precision.
For instance, electroencephalography (EEG) and functional magnetic resonance imaging (fMRI) have paved the way for real-time brain activity tracking. As a result, we now have tools that can interpret complex cognitive processes, potentially revolutionizing fields as varied as education, rehabilitation, and user experience design.
"As we integrate these sensors into various domains, it becomes essential to develop a framework that not only maximizes benefits but also safeguards against ethical infringements."
Prolusion to Brain Activity Sensors
Understanding brain activity sensors is crucial as they bridge the gap between our mental processes and technological capabilities. For researchers and students alike, these sensors unveil new insights into how our brain operates, making it a topic of immense interest in both academic and applied contexts. The drive for enhancement in diagnosis and treatment of neurological conditions has escalated the significance of these devices, pushing them from theoretical realms into practical applications.
Definition and Scope
Brain activity sensors are instruments designed to measure and interpret neural activity. They encompass a range of technologies, each with its own methods and specific applications. At their core, they aim to decode the electrical impulses, magnetic fields, or metabolic changes associated with brain functions. The instruments—ranging from Electroencephalography to fMRI—have become pivotal in fields like psychology, cognitive neuroscience, and medicine, enabling us to monitor brain functions in real time.
Such sensors not only provide a window into cognitive processes but also have a direct impact on developing therapies for various ailments. The scope extends beyond research, penetrating areas like gaming and human-computer interaction, emphasizing their cross-disciplinary relevance. This intersection of technology and neuroscience challenges our understanding of consciousness and perception, making it imperative to dive deeper into this topic.
Historical Context
Tracing the roots of brain activity sensors takes us back to the mid-20th century when researchers first began exploring how to visualize and measure brain functions. Early experiments in psychology utilized rudimentary devices, but the real turning point came with the advent of technologies like Electroencephalography in the 1920s, marking the first attempt at measuring electrical activity in the brain.
Subsequent advancements in neuroimaging, particularly with the development of functional Magnetic Resonance Imaging in the 1990s, revolutionized how we perceive brain activity. These technologies not only opened the doors for a plethora of research studies but also led to significant breakthroughs in understanding mental health conditions. Today, brain activity sensors continue to grow, merging innovations from artificial intelligence, data science, and neuroscience.
"The journey from the first EEG to today's advanced imaging techniques shows a remarkable evolution in our capacity to study the brain".
In summary, the historical context provides a backdrop against which the current sophistication of brain activity sensors can be appreciated. As we navigate this landscape, understanding both its evolution and technological advancements fuels a broader comprehension of the human experience.
Types of Brain Activity Sensors
The realm of brain activity sensors is diverse and continuously evolving. Understanding the various types of sensors is crucial for harnessing their potential in both clinical and research settings. Each type serves a specific need and offers unique benefits. From diagnosing mental health issues to steering the development of immersive technologies, these sensors play a pivotal role in unraveling the complexities of the human brain. Let's explore some of the most significant types of brain activity sensors in detail.
Electroencephalography (EEG)
Electroencephalography, commonly referred to as EEG, is a technique that measures the electrical activity of the brain. Through a series of electrodes placed on the scalp, EEG captures changes in neural activity, providing insights into different cognitive states. The ability to record brainwaves in real-time makes it invaluable in both clinical and experimental environments.
One of the key advantages of EEG is its high temporal resolution. This allows researchers to observe brain activity as it happens, offering a clearer picture of how the brain responds to stimuli or processes information. EEG is commonly used in diagnosing epilepsy and sleep disorders, highlighting its clinical significance.
However, while EEG excels in timing precision, it lacks spatial resolution, rendering it less effective in pinpointing the exact origin of the electrical activity within the brain. This limits the sensor's capability somewhat, making it essential that researchers weigh the pros and cons when selecting this method for their specific needs.
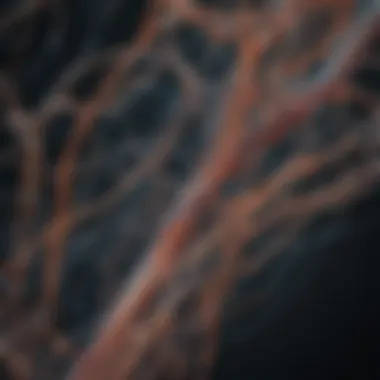
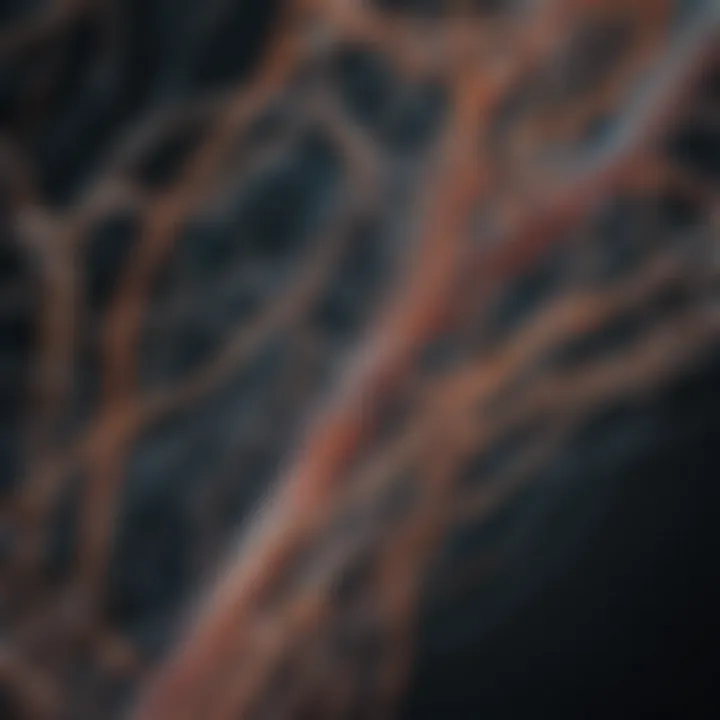
Functional Magnetic Resonance Imaging (fMRI)
Functional Magnetic Resonance Imaging is another leading tool in the toolkit of neuroscience. Unlike EEG, fMRI operates on the principle of measuring blood flow in the brain, which correlates with neural activity. When a particular area of the brain is more active, it requires more oxygen. fMRI detects these changes, allowing for a detailed mapping of brain functions.
Because of its superior spatial resolution, fMRI can pinpoint the exact location of brain activity, making it an excellent choice for exploring brain anatomy and functions. This capacity has led to its frequent use in cognitive research and clinical diagnosis, particularly for assessing conditions such as Alzheimer's disease and brain tumors.
While fMRI delivers clear visuals of brain activity, it comes with some drawbacks. The slower temporal resolution means it cannot capture rapid changes in brain activity as effectively as EEG can. This trade-off makes it necessary for researchers to consider the specific focus of their studies when opting for fMRI.
Magnetoencephalography (MEG)
Magnetoencephalography stands apart as a powerful technique for measuring the magnetic fields generated by neural activity. This method offers an enticing combination of both high spatial and temporal resolution. MEG can detect dynamic brain processes, making it suitable for real-time monitoring of brain functions.
The precise localization of brain activity afforded by MEG enables researchers to observe the functioning of various brain networks, leading to insights that are pivotal in domains such as cognitive neuroscience and neurosurgery. Notably, MEG is utilized in the pre-surgical mapping of functions to minimize the risk of damages.
One significant caveat of this technology is its cost, which can be a limiting factor for widespread application. This means that while MEG provides compelling data, not every facility can afford to utilize this remarkable technology.
Near-Infrared Spectroscopy (NIRS)
Near-Infrared Spectroscopy presents a distinctive approach that relies on light to assess changes in oxygenated and deoxygenated hemoglobin in the brain tissue. It's advantageous in its non-invasive nature and relative ease of use. NIRS is particularly valuable for examining brain activity in infants and young children, where traditional methods like fMRI and EEG might pose challenges.
Though it provides a balance between temporal and spatial resolution, NIRS tends to be more limited in depth penetration. The signals it gathers might only reflect activity in superficial brain tissues, which is a decisive consideration when older children or adults are in focus.
Moreover, NIRS has gained traction in the field of wearable technology. This allows for real-time monitoring in naturalistic settings, proving beneficial in a wide array of applications, including sports science and even commercial products designed for mental wellness.
How Brain Activity Sensors Work
Understanding how brain activity sensors operate is essential for fully grasping their implications in various disciplines, ranging from medicine to technology. These sensors are not just sophisticated gadgets; they are powerful tools that open doors to understanding our cognitive landscape. They capture the electrical and magnetic signals from the brain, offering insight into everything from how we think to how we feel.
Principles of Functionality
Brain activity sensors rely on several scientific principles to function effectively. At their core, they are designed to measure the activity of neurons. When a neuron fires, it creates a tiny electrical change, which can be detected using various technologies.
Electroencephalography (EEG), for instance, uses electrodes placed on the scalp to capture these electrical signals. It picks up the brain's activity in real-time, enabling researchers and clinicians to observe brain processes as they unfold. This is crucial in clinical settings, where understanding the timing and nature of brain activity can lead to better diagnoses and treatments.
In contrast, functional magnetic resonance imaging (fMRI) takes a different approach. It measures changes in blood flow, using the concept that active brain areas require more oxygen. This non-invasive method allows for the visualization of brain activity over prolonged periods, which is not possible with EEG due to its limited temporal resolution.
Thus, various sensors deploy distinct methods to gather valuable data. Each has its unique strengths, tailored to specific research or clinical objectives. The choice of sensor depends mainly on the type of information needed—whether it's fast, moment-to-moment fluctuations in brain activities or the more complex spatial distribution of brain functions.
Data Acquisition and Processing
Once the signals are captured, the next step is data acquisition, which involves converting physical signals into a format that can be analyzed. For example, EEG devices convert brain signals into waveforms that indicate different brain states—like sleep, attention, or alertness. This transformation process is fundamental since direct interpretation of the raw signals isn’t meaningful without proper context and processing.
Data Processing Steps:
- Preprocessing: Filters out noise from the data, ensuring that the actual brain signals are clear and usable.
- Feature Extraction: Identifies key characteristics of the signals, such as frequency bands that correspond to specific mental states.
- Analysis: Employs statistical methods and algorithms to interpret the data. Machine learning techniques are increasingly integrated into this phase, enabling improved analysis and insights.
The processing of brain activity data is often complex and can take substantial time. As tools become more advanced, the efficiency of data processing continues to improve, allowing for real-time analysis in many applications.
“The real beauty of brain activity sensors lies in their transformational ability; they not only collect data but also tell stories about our thoughts and feelings.”
These sensors have indeed changed the game in fields like clinical psychology, where they help visualize mental states and facilitate neurofeedback therapy. For researchers, they uncover how complex brain systems interconnect, driving forward our understanding of not just the brain, but what it means to be human.
As we continue to innovate and explore further, the fundamental principles and processing techniques behind these sensors will play a crucial role in their development and utilization.
Applications of Brain Activity Sensors
Understanding the diverse applications of brain activity sensors is crucial as it sheds light on how these devices are transforming various fields, especially in medicine, cognitive research, and human-computer interaction. Their ability to capture and analyze neural activities offers unprecedented insights and practical solutions to complex issues, redefining the way we approach health, cognitive science, and technology integration. Let's explore these applications more closely.
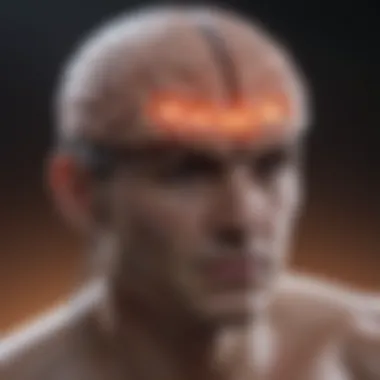
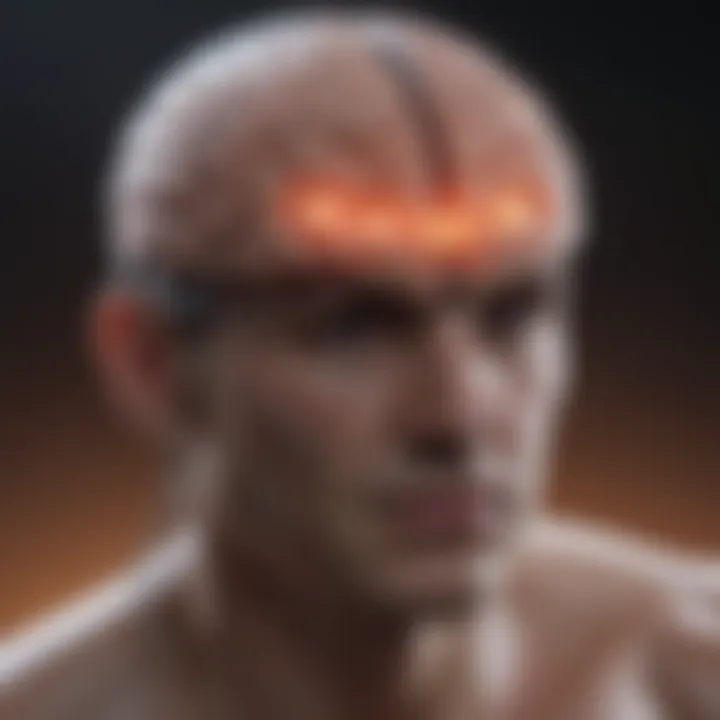
Clinical Uses in Medicine
Neurofeedback Therapy
One of the standout applications in the medical field is neurofeedback therapy. This technique involves training individuals to alter their brain activity through real-time feedback, often achieved via EEG devices. The process can be quite sophisticated—it’s not merely a case of looking at numbers; individuals learn to modulate the activity in specific brain regions. The key characteristic of neurofeedback is its focus on self-regulation, making it a popular choice for conditions such as ADHD, anxiety, and depression.
The unique feature of neurofeedback is that it empowers patients to acquire cognitive control over their stimuli responses. This autonomy can foster a sense of agency in treatment, which can be greatly motivating. However, a downside exists—its effectiveness often relies on the individual’s engagement and the skill of the clinician, which can vary significantly.
Seizure Detection
Another vital clinical use is seizure detection. These systems, employing techniques like EEG and wearable sensors, monitor brain activity continuously to identify patterns indicative of seizure onset. The main highlight here is their ability to provide timely alerts to patients or caregivers, possibly preventing accidents or severe episodes. This technology is a lifeline for many patients living with epilepsy.
What makes seizure detection unique is its capability for real-time response. This means that individuals can receive immediate assistance when needed most. However, the technology isn’t without its complications; false positives can occur, leading to unnecessary distress or intervention, raising questions about reliability and trust in such systems.
Mental Health Monitoring
Mental health monitoring through brain activity sensors is an emerging arena. By tracking changes in brainwaves, clinicians can gain valuable insights into conditions like depression and bipolar disorder. The primary advantage lies in its non-invasiveness and the wealth of data it provides, which can lead to more informed treatment plans.
The unique feature of mental health monitoring is its potential for proactive intervention. Identifying subtle shifts in brain activity could lead to earlier diagnosis and better treatment outcomes. On the flip side, the ethics surrounding data collection is a concern; patients may worry about privacy and how their data is used.
Cognitive Research and Neuroscience
Understanding Neural Pathways
In the realm of cognitive research, understanding neural pathways is paramount. Brain activity sensors help researchers map the intricate webs of connections that influence thoughts, memories, and behaviors. This is significant for developing treatments for cognitive impairments and enhancing our grasp of brain functionality.
The key characteristic of this application is its contribution to elucidating how different regions of the brain communicate. This can lead to breakthroughs in understanding and treating neurological disorders. However, the challenge lies in the complexity of the brain itself—interpreting data from these sensors requires extensive expertise and can yield ambiguous results.
Cognitive Load Assessment
Cognitive load assessment is another significant application. By measuring how much mental effort a person exerts during tasks, researchers can better understand various cognitive processes. This application is particularly relevant in educational settings and ergonomics.
The unique feature of cognitive load assessment is its direct insight into how individuals process information. This can lead to designing more effective learning environments or improving user experiences in tech. Yet, the challenge is in accurately translating data into actionable insights, as cognitive processes can be influenced by numerous variables.
Human-Computer Interaction
Brain-Computer Interfaces
Brain-computer interfaces are pushing the boundaries of how we interact with technology. By translating brain signals into commands, these systems allow users to control devices, potentially leading to revolutionary applications in assistive technologies for individuals with disabilities.
The key characteristic of this technology is its capacity for direct communication between brain and machine, which can be incredibly liberating for users. However, the complexities involved in interpreting brain signals can lead to limitations in effectiveness, with accuracy and speed being critical factors that need continual improvement.
Augmented Reality Applications
Lastly, augmented reality applications are increasingly integrating brain activity sensors to enhance user experiences. By understanding user emotions and cognitive responses, AR can be tailored to fit individual preferences, creating more immersive environments.
The unique feature here is the ability to adapt in real-time to a user’s mental state. This not only enriches the experience but also fosters a deeper connection between the user and the technology. Nonetheless, developing these applications involves substantial technical challenges, particularly in achieving seamless integration and data processing.
Brain activity sensors are not just tools; they are gateways to new understandings of the brain and its functions.
In summary, the applications of brain activity sensors span a wide range of fields, with the potential to revolutionize medical treatments, enhance cognitive research, and redefine human-computer interaction, making them a crucial focus in both current and future studies.
Ethical Considerations in Brain Activity Sensing
The rise of brain activity sensing technologies brings to the forefront numerous ethical concerns that warrant careful consideration. These sensors have the potential to unlock secrets of the human mind, but with great power comes significant responsibility. As we strive to seek knowledge and improve our understanding of cognitive processes, it is vital to navigate the murky waters of ethics. Framing our discussions around the implications of brain activity sensors adds significant depth to the current narrative in neuroscience and technology.
With applications spanning from clinical uses to entertainment, the ethical landscapes of privacy and consent loom large. Essentially, the integration of technology with human data necessitates robust discussions that examine how we protect individuals while advancing scientific inquiry.
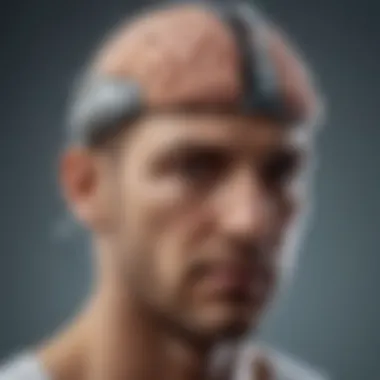
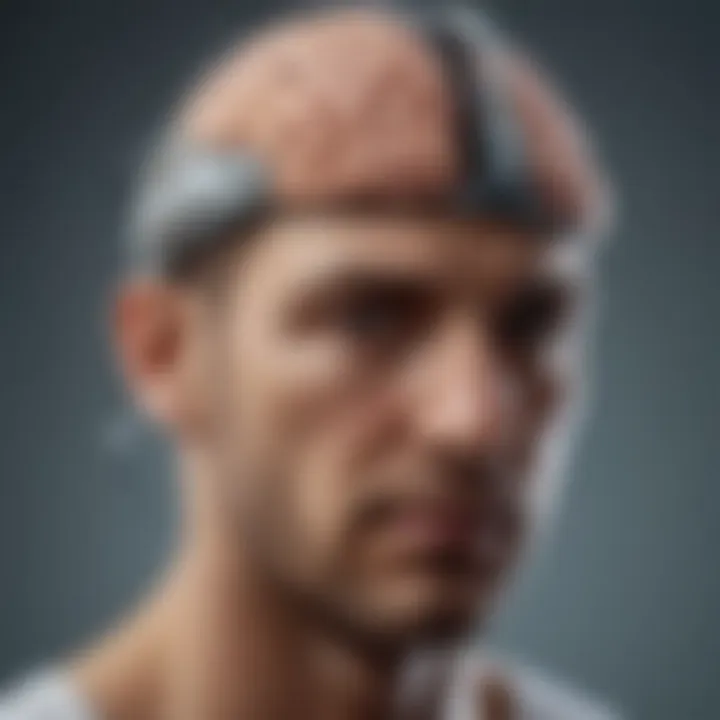
Privacy Issues
In this digital age, privacy can feel like a faint whisper amidst the noise of technological advancement. Brain activity sensors operate by collecting sensitive neural information, which raises the specter of breaches that could invade personal mental sanctuaries. Imagine having your thoughts, emotions, or cognitive states analyzed without your explicit permission. This is not science fiction but a real concern that requires attention.
With data collection methods becoming increasingly sophisticated, malicious intent could exploit vulnerable data streams. Protecting user data must be at the forefront of the developers' minds. There are several aspects worth considering:
- Data Security: It’s crucial that developers implement stringent measures to safeguard collected information. Unauthorized access could lead to not only data breaches but also emotional harm to individuals if sensitive information were to be disclosed.
- Ownership: Who owns the data harvested from brain activity sensors? This question lingers, as individuals often relinquish their ownership rights upon agreeing to end-user agreements without thorough understanding.
- Surveillance: The potential usage of this technology for surveillance purposes is an alarming possibility where mental data may be used for unwanted scrutiny or manipulation.
Informed Consent
Turning attention to informed consent, it’s essential to remember the principle that individuals should be fully aware of what they’re agreeing to when they take part in research or other activities involving brain activity sensors. Imagine signing a waiver without understanding the consequences; it would feel like stepping into a minefield blindfolded.
Informed consent shouldn’t just be a box to tick off; it must be a comprehensive process that empowers participants. Here are some key considerations related to informed consent in brain activity sensing:
- Clarity in Communication: Researchers must present information in clear, jargon-free language that allows individuals of varying backgrounds to understand risks and benefits fully.
- Ongoing Consent Process: Consent shouldn’t be static. As studies progress or new technologies are introduced, a continuous dialogue regarding consent can ensure participants remain informed and comfortable.
- Withdrawal Options: Participants should have the right to withdraw their consent at any time. This should be as easy as pie, enabling an exit from the study without any repercussions.
In closing, ethical considerations represent the foundational bedrock for the development and deployment of brain activity sensing technologies. Addressing privacy concerns and ensuring informed consent can forge a path towards responsible innovation, ensuring that technology serves humanity rather than threatens it.
Future Directions in Brain Activity Sensing Research
The exploration of brain activity sensors is at a pivotal point, and understanding the future directions of this research is crucial. The ongoing advancements in technology and the shifting landscape of neuroscience present numerous opportunities. As these sensors evolve, they promise not only to enhance current applications but also to open avenues for innovations that could reshape our approach to understanding the brain.
Technological Innovations
Technology is the lifeblood of progress in brain sensing. One significant innovation on the horizon is the development of portable and wearable devices that capture brain activity without the bulk of traditional equipment. Imagine a headband that you might wear while simply watching TV, capturing your brain's response to various stimuli. Such a device would dramatically change how we collect data in real-world scenarios.
Moreover, advancements in machine learning are set to play a critical role in data analysis. With algorithms that can learn from vast amounts of brain sensor data, researchers could potentially identify patterns and correlations that were previously invisible. This could lead to breakthroughs in understanding conditions like epilepsy or mood disorders.
"Wearable tech is not just a trend; it is reshaping how we engage with our own minds."
Additionally, the integration of brain sensors with biofeedback mechanisms might offer tailored therapeutic options. For example, a person receiving neurofeedback for anxiety could be able to see real-time data on their brain's activity while employing relaxation techniques. This connection could empower individuals to make immediate adjustments based on their cognitive responses.
Cross-disciplinary Collaborations
As the field of brain activity sensing matures, the need for collaboration across disciplines becomes ever more apparent. Neuroscience, engineering, psychology, and even ethics must converge to maximize the benefits and mitigate the risks associated with these technologies. For instance, when designing sensors, engineers need insights from neuroscientists on brain function to create effective devices.
Furthermore, collaboration can lead to hybrid fields, marrying the insights of cognitive science with advances in artificial intelligence. Researchers from different domains can share perspectives that enrich the development of more accurate and sophisticated brain sensing technologies. A psychologist might provide insights on behavioral factors that inform sensor design, while an ethicist can guide safe implementation standards.
Incorporating interdisciplinary training for students and professionals in these fields will also be essential. Establishing forums, workshops, and research networks can stimulate the sharing of knowledge and foster innovation.
As brain activity sensors propel forward, their future hinges on these partnerships, opening possibilities that stretch the bounds of what we currently perceive as attainable. The capacity to share expertise not only enhances the research but also ensures that the societal implications of these technologies are thoroughly understood and addressed.
The End
The realm of brain activity sensors offers vast potential, shaping our understanding of both neuroscience and technology. This article traversed through various key aspects, exposing the intricacies behind how these sensors operate and their diverse applications.
Summary of Key Points
A few of the essential highlights from this discussion include:
- Types of Sensors: We explored a range of brain activity sensors like Electroencephalography and Functional Magnetic Resonance Imaging, demonstrating how each sensor provides unique insights into brain activity.
- Mechanisms and Functionality: The discussion covered the principles of operation for different sensors, offering a glimpse into data acquisition and processing.
- Applications Across Fields: Clinical uses in medicine, cognitive research, and advancements in human-computer interaction showcased the versatile applications of these technologies.
- Ethics and Privacy: We reflected on the ethical dimensions surrounding brain sensors, such as the need for informed consent and addressing privacy concerns.
- Future Directions: The article also hinted at potential future innovations in technology, urging interdisciplinary collaboration for more effective brain activity sensing methods.
This article concisely highlighted these focal points, setting the stage for further discussion and inquiry into how brain activity sensors may redefine numerous fields.
Implications for Future Research
Looking ahead, there are numerous avenues for exploration within this field. The insights garnered from brain activity sensors hold potential benefits and challenges for future research initiatives:
- Technological Integration: As sensors evolve, integrating emerging technologies like artificial intelligence and machine learning can enhance data analytics. One can expect advanced algorithms to improve our understanding of cognitive processes.
- Expanded Clinical Applications: There is a need for more research focusing on innovative ways to utilize brain sensors in mental health therapies, possibly leading to tailored treatments based on real-time brain activity.
- Ethical Frameworks: The conversation on ethics must keep pace with technological advancements. Developing robust ethical guidelines will be crucial to navigate privacy concerns and informed consent complexities as brain activity sensing technology becomes ubiquitous.
- Collaboration Across Disciplines: Collaborations among neuroscientists, technologists, and ethicists can lead to well-rounded solutions, ensuring that the development of such technologies aligns with societal values and ethical standards.
In summary, the evolving landscape of brain activity sensors presents both exciting possibilities and pressing challenges. The ongoing discourse on their implications will undoubtedly inform future advancements, shaping how we comprehend the mind and its connection to technology.
"As the line between human cognition and machine interaction blurs, understanding the nuances of brain activity becomes more essential than ever."