Innovative Approaches in Positron Cancer Treatment
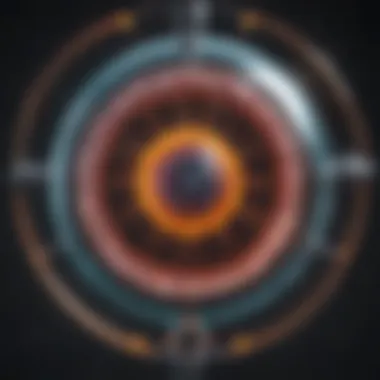
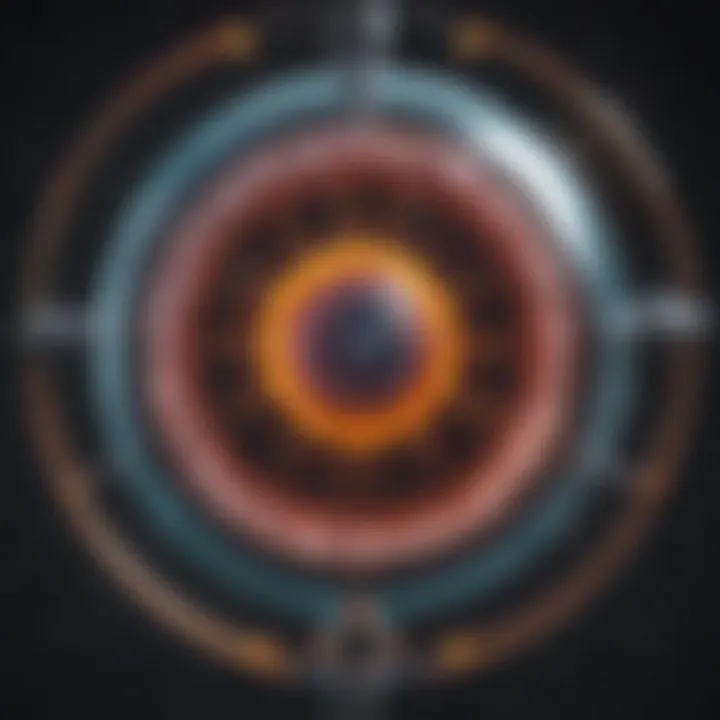
Intro
The landscape of cancer treatment is rapidly evolving, driven by technological advancements and innovative methodologies. Among these pioneering approaches, positron emission tomography (PET) has garnered significant attention. This imaging technique, coupled with radiopharmaceuticals, is revolutionizing diagnosis and personalized treatment in oncology. Understanding the implications of these advancements is critical for students, researchers, educators, and professionals involved in the field.
In this exploration, we will delve into the key points surrounding positron cancer treatment, including its scientific principles, practical applications, and the ongoing challenges faced within this domain. We will also discuss the integration of PET in treatment planning and its role as a diagnostic tool. Overall, this comprehensive guide aims to enhance knowledge of state-of-the-art techniques and their relevance in improving patient outcomes.
Prelude to Positron Cancer Treatment
Positron cancer treatment represents a significant advancement in cancer care. Its roots in physics and medical imaging have led to enhanced methods of diagnosis and therapy. Understanding the mechanisms of positron emission can offer valuable insights for oncologists, researchers, and healthcare professionals involved in cancer treatment.
This section elucidates the importance of positronic techniques in modern oncology and highlights their impact on treatment strategies. Specifically, it focuses on the benefits of accurate diagnosis, treatment planning, and the integration of radiopharmaceuticals. Understanding these aspects can improve patient outcomes and foster personalized treatment approaches, which are central to the future of oncological care.
Understanding Positrons
Positrons are the antiparticles of electrons and are significant in the field of nuclear medicine. When a positron encounters an electron, they annihilate each other, resulting in the emission of photons, usually in the form of gamma rays. This phenomenon is central to positron emission tomography (PET) imaging, a tool that enables detailed visualization of metabolic processes in the body.
Positrons are generated from certain radionuclides used in imaging. For example, fluorine-18, commonly used in oncological PET scans, decays by emitting positrons. The detection of these emitted gamma rays helps create high-resolution images of cancerous tissues, allowing for earlier diagnosis and the formulation of more effective treatment plans.
Overview of Cancer Treatment Modalities
Cancer treatment is not monolithic; it encompasses a variety of modalities. These include surgery, radiation therapy, chemotherapy, and more recent innovations like immunotherapy and targeted therapies. Each method has its advantages and limitations, depending on the cancer type and stage.
Radiotherapy utilizes ionizing radiation to target and destroy cancer cells. Chemotherapy employs drugs that kill rapidly dividing cells, often leading to side effects due to the lack of specificity. Immunotherapy taps into the body’s immune system, offering personalized treatment pathways based on individual tumor characteristics.
Emerging treatments like precision medicine and personalized therapy are gaining traction. These involve tailoring treatment strategies based on a patient’s genomic information, which fundamentally shifts the paradigm of oncology. Positron-based techniques fit well within this framework, as their precise imaging capabilities can guide tailored therapy decisions.
The Science Behind Positron Emission
Understanding the science behind positron emission is crucial for comprehending how positron-based techniques are applied to cancer treatment. This section delves into two significant facets: the theoretical foundations of positron emission tomography and the mechanics of how positrons interact with matter. Both areas are pivotal in enhancing diagnostic accuracy and treatment efficacy, contributing to improved outcomes for oncology patients.
Theoretical Foundations of Positron Emission Tomography
Positron Emission Tomography, or PET, is a revolutionary imaging technique that utilizes the properties of positrons to visualize metabolic processes in the body. The core principle rests upon the annihilation event when a positron, which is the antiparticle of an electron, interacts with an electron. This annihilation leads to the emission of two gamma photons, which travel in opposite directions. Detecting these photons allows for the reconstruction of images that represent the distribution of radioactive tracers within the body.
The underlying physics of PET is rooted in nuclear medicine, where radiopharmaceuticals containing positron emitters like Fluorine-18 are utilized. These compounds are administered to the patient and accumulate in particular tissues based on their metabolic activity. This targeted distribution highlights areas of abnormal activity, often indicative of tumors or other pathological conditions. Thus, understanding the theoretical underpinnings of PET is essential as it informs clinicians about potential tumor locations, shapes, and metabolic characteristics, guiding further diagnostic and therapeutic steps.
Mechanics of Positron Interactions with Matter
Positrons interact with surrounding matter primarily through ionization and annihilation processes. When a positron is emitted by a radionuclide, it travels a short distance before losing energy through interactions with electrons in the surrounding tissue. This energy loss leads to ionization, creating pairs of ions and free electrons, which can cause biological damage. However, these interactions are generally localized due to the short range of the emitted positrons, making them suitable for imaging and targeted therapy applications.
Upon losing sufficient energy, the positron eventually encounters an electron, resulting in annihilation. This conversion of mass into energy produces two gamma photons. These photons, traveling at high speeds, escape the body and are detected by the PET scanner, allowing for image reconstruction.
Understanding these mechanics is imperative for improving imaging techniques and therapeutic interventions. Recognizing how positrons interact with the biological environment helps in optimizing radiation doses and enhancing the safety of procedures, ultimately benefiting patients.
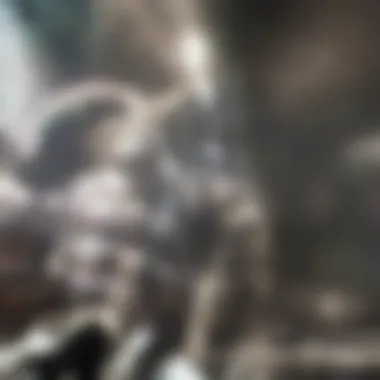
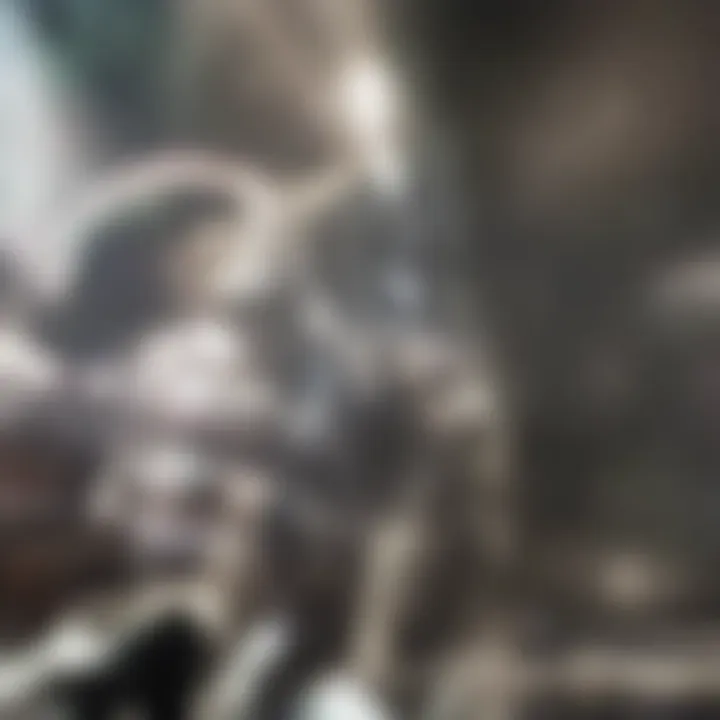
Effective applications of positron interactions can significantly minimize unwanted side effects while maximizing diagnostic precision and treatment effectiveness.
Clinical Applications of Positron-Based Techniques
The integration of positron-based techniques in clinical oncology has revolutionized how cancer is diagnosed and treated. The clinical applications of these techniques offer precision, improving treatment outcomes while minimizing harm to healthy tissues. Understanding these applications is crucial for oncologists, researchers, and healthcare practitioners aiming to tailor therapies for individual patients.
Positron Emission Tomography in Oncology
Positron Emission Tomography, or PET, is a critical tool in modern oncology. It employs radiolabeled glucose analogs to visualize metabolic activity in tumors. This imaging technique allows for early detection of cancer as it identifies abnormal cellular metabolism associated with malignancies. In contrast to traditional imaging methods, PET provides a functional view of cancerous tissues, which is invaluable in both diagnosis and treatment planning.
The benefits of PET in oncology include:
- Early Detection: Identifies cancer at stages where treatment can be more effective.
- Staging and Treatment Planning: Offers insights into tumor spread, guiding surgical and therapeutic decisions.
- Assessing Treatment Response: Helps evaluate how well a treatment is working, allowing for timely adjustments.
Despite its advantages, the use of PET must be approached with caution. The high costs of radiopharmaceuticals and the need for specialized equipment can limit accessibility in some regions. Furthermore, the interpretation of PET scans requires expertise to reduce the risk of misdiagnosis, which can lead to inappropriate treatment decisions.
Radionuclide Therapy and Its Applications
Radionuclide therapy represents another pivotal advancement in positron cancer treatment. This form of treatment utilizes radioactive substances to target cancer cells directly. Common radionuclides used include Iodine-131 for thyroid cancer and Lutetium-177 for neuroendocrine tumors. This therapy can provide effective outcomes, particularly in cases where conventional methods fall short.
The applications of radionuclide therapy in oncology can be categorized as follows:
- Targeted Treatment: Directly delivers radiation to tumor cells, minimizing damage to surrounding healthy tissue.
- Palliative Care: Offers relief from symptoms in advanced cancer, significantly improving the quality of life for patients.
- Combination Therapy: Often used in conjunction with other treatment modalities such as chemotherapy or radiotherapy to enhance overall efficacy.
However, there are important considerations to keep in mind with radionuclide therapy. Dosimetry must be meticulously calculated to ensure optimal radiation exposure. Safety is also paramount, necessitating strict protocols to protect both patients and healthcare professionals from radiation exposure.
"The clinical application of positron-based techniques in oncology represents a pivotal shift towards more personalized and effective cancer care."
In summary, the clinical applications of positron-based techniques are essential to advancing oncology. Understanding their potential and limitations encourages improved patient outcomes and tailors therapeutic approaches to meet individual needs.
Radiopharmaceuticals in Positron Cancer Treatment
Radiopharmaceuticals are pivotal in positron cancer treatment, serving as essential tools for both diagnostic imaging and therapeutic applications. Their role is to enhance the efficacy of cancer treatment by providing targeted delivery of radiation to malignant tissues. This specificity allows for minimizing exposure to surrounding healthy tissues, which is crucial in improving patient safety and treatment outcomes.
The use of radiopharmaceuticals encompasses various forms, each offering distinct advantages depending on the cancer type and treatment goals. In addition to enhancing precision, these agents facilitate real-time imaging during treatment, allowing oncologists to monitor the response and adjust treatments as needed.
Types of Radiopharmaceuticals Used
The spectrum of radiopharmaceuticals utilized in positron cancer treatment includes a variety of agents, most commonly radiolabeled compounds that emit positrons.
A few notable examples include:
- Fluorodeoxyglucose (FDG): Widely used in positron emission tomography (PET) scans, FDG mimics glucose and is preferentially taken up by cancer cells, thus facilitating tumor imaging and assessment.
- Gallium-68 and Lutetium-177: Used for both diagnostics and treatment, particularly in neuroendocrine tumors, enhancing imaging capabilities as well as treatment efficacy.
- Carbon-11 and Nitrogen-13: These isotopes assist in studying metabolic activities of tumors at a molecular level, providing insights into tumor behavior.
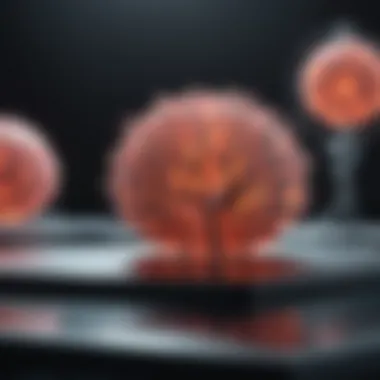
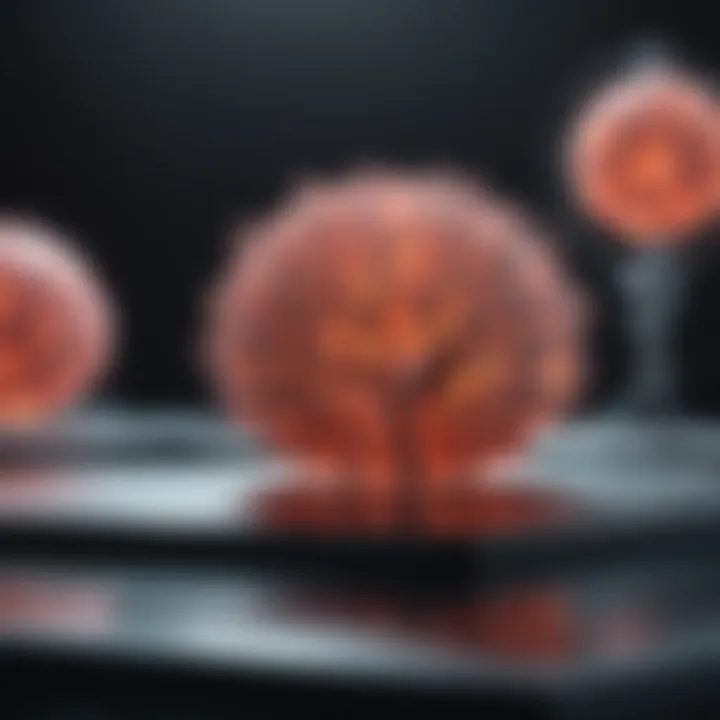
The choice of radiopharmaceutical often hinges on the type of cancer and the specific metabolic characteristics of the tumor cells, allowing for a tailored approach to treatment.
Dosimetry and Safety Considerations
Dosimetry refers to the calculation and assessment of the radiation dose received by the patient. In the context of positron cancer treatment, accurate dosimetry is crucial for ensuring that the therapeutic effects are maximized while minimizing potential side effects.
Safety considerations include:
- Radiation Exposure: Understanding the amount and type of radiation a patient is exposed to is vital for risk assessment and management.
- Individual Variability: Patients may exhibit different biological responses to radionuclide therapy, necessitating personalized dosimetry calculations that account for factors like age, weight, and overall health status.
- Regulatory Regulations: Compliance with national and international safety standards is essential. This helps ensure that radiopharmaceuticals are administered safely and effectively during treatment.
In summary, the integration of robust dosimetry protocols and safety considerations into radiopharmaceutical use significantly contributes to the overall success of positron cancer treatment strategies. By focusing on these elements, oncologists can enhance patient safety and treatment efficacy, optimizing outcomes in cancer care.
Technological Innovations in Imaging
The field of positron cancer treatment has seen substantial advancements in imaging technologies. These innovations not only enhance diagnostic capabilities but also enable accurate treatment planning and monitoring. Imaging is crucial for understanding tumor biology, determining staging, and assessing treatment efficacy. The integration of new technologies increases the precision of positron emission tomography (PET) and its complementary imaging modalities.
Enhancements in PET Scanner Technology
Recent developments in PET scanner technology are noteworthy. Modern machines have improved spatial resolution, which leads to finer imaging quality. This is vital, as tumors can be very small, and capturing their details is essential for effective treatment. Enhanced sensitivity allows for the detection of lower concentrations of radiopharmaceuticals, helping in identifying malignancies at earlier stages.
Another important innovation is the combination of time-of-flight (TOF) imaging. This advanced method optimizes signal detection by measuring the time it takes for positrons to collide with electrons. As a result, the images generated are not only more accurate but also clearer. This helps oncologists to precisely locate tumors, which is critical for tailoring treatment options.
The introduction of digital PET systems is also significant. These systems utilize digital photomultiplier technology, which improves overall image quality and data acquisition speed. This reduces the time patients spend in the scanner while still maintaining high diagnostic accuracy. Furthermore, the reduced noise in digital systems enhances the interpretation of images, aiding in better clinical decision-making.
- Key benefits of newer PET scanner technologies:
- Higher resolution and sensitivity
- Faster scanning times
- Improved image quality
- Enhanced diagnostic accuracy
Integration with MRI and CT Scans
The integration of positron emission tomography with magnetic resonance imaging (MRI) and computed tomography (CT) represents a significant advancement in the imaging landscape. This convergence allows for a more holistic view of the cancerous tissues. By combining the functional imaging capabilities of PET with the anatomical detail provided by MRI and CT, healthcare providers can gather comprehensive data about the tumor.
This triad of imaging techniques enhances the understanding of tumor metabolism, morphology, and surrounding structures. For instance, MRI excels in soft tissue contrast and does not expose patients to ionizing radiation, making it an excellent partner for PET. This combination leads to more accurate staging of cancers and better assessment of treatment response.
Moreover, the fusion of these imaging modalities facilitates personalized treatment plans. Oncologists can tailor interventions based on detailed understanding derived from this multi-faceted imaging approach. This level of detail can help highlight areas of treatment resistance, guiding future therapeutic strategies.
"The multidisciplinary approach incorporating PET, MRI, and CT has revolutionized the way we visualize and treat cancer, underscoring the critical role of technological innovation in oncology."
Challenges in Positron Cancer Treatment
Understanding the challenges in positron cancer treatment is vital for advancing both research and clinical practices. While positron emission tomography and related techniques show promise, there are specific hurdles that need careful consideration. Recognizing these challenges is crucial because they can significantly influence treatment outcomes and the pathway to future advancements. This section will explore several limitations and patient-specific factors that often complicate currently available techniques.
Limitations of Current Techniques
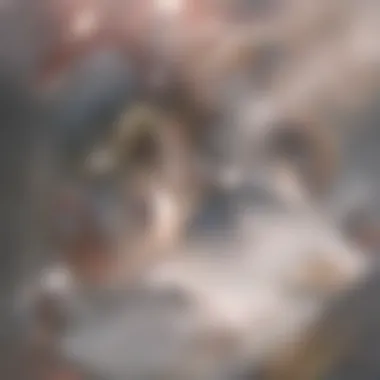
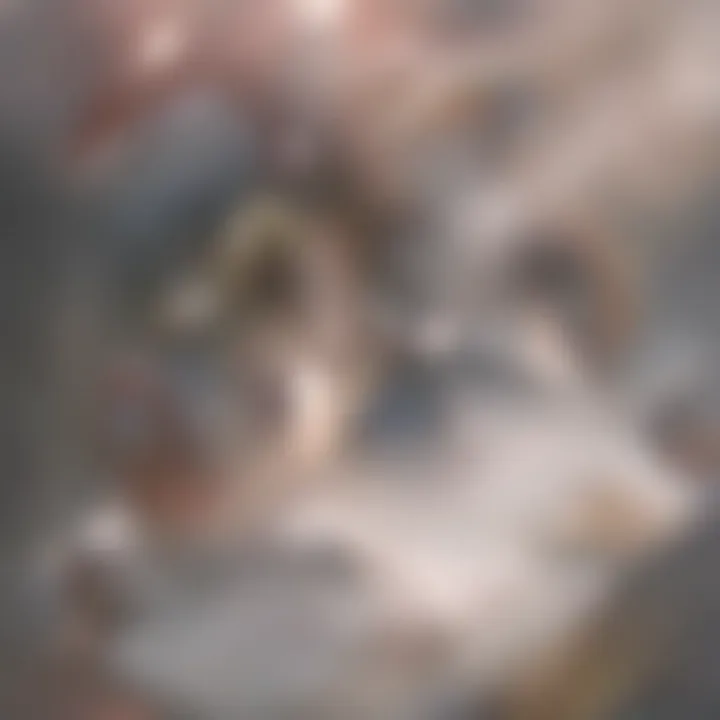
Positron cancer treatment relies heavily on technology for both diagnosis and treatment planning. However, there are inherent limitations that affect its effectiveness.
- Sensitivity and Specificity: Current techniques, while advanced, may still struggle with sensitivity and specificity in detecting certain types of tumors. False positives can lead to unnecessary anxiety and procedures, whereas false negatives can delay necessary treatment.
- Radiopharmaceutical Limitations: The effectiveness of radiopharmaceuticals varies. Factors such as the tumor's biochemistry and accessibility can affect how well these drugs perform. Some tumors may not uptake the radionuclide effectively, leading to inadequate treatment outcomes.
- Technical Proficiency: The precision of treatment execution relies on the technical capability of medical professionals and quality of the equipment. Variability in expertise can lead to differences in patient outcomes.
- Costs and Accessibility: High costs of evaluating and treating patients with positron techniques can limit access to these technologies, particularly in under-resourced settings. This raises concerns about equity in cancer treatment options.
"Understanding the limitations of current techniques is essential for refining future approaches in positron-based cancer therapies."
The limitations above call for rigorous research and development to overcome these challenges and improve the efficacy of positron cancer treatment methods.
Addressing Patient-Specific Factors
Each patient presents a unique set of circumstances that can influence their response to positron cancer treatment. Recognizing and addressing these individual factors is critical in enhancing treatment effectiveness.
- Genetic Variability: Individual genetic profiles can impact how patients metabolize and respond to radiopharmaceuticals. Personalized medicine approaches need to take these differences into account to optimize treatment plans.
- Tumor Characteristics: Variability in tumor biology, such as size, location, and the presence of necrosis or fibrosis, can affect treatment responses. Personalized imaging and dosing strategies may be necessary for different tumor types.
- Comorbid Conditions: Patients often present with comorbidities that can complicate treatment. These factors must be thoroughly evaluated to ensure safe and effective use of positron-based therapies.
- Psychosocial Elements: The emotional and psychological state of patients can also play a role. Understanding these aspects is important for creating a supportive environment that promotes adherence to treatment plans.
By focusing on patient-specific variables, the field can evolve towards more tailored treatments that better address the complexities of individual cases. This approach holds promise for improving overall patient outcomes and satisfaction in positron cancer treatment.
Future Directions in Positron Cancer Treatment
As the field of oncology continues to evolve, future directions in positron cancer treatment hold significant promise. The integration of advanced technologies and innovative approaches can enhance diagnostic accuracy and treatment efficacy. Understanding these future directions is crucial for healthcare professionals seeking to harness the full potential of positron-based techniques in delivering targeted therapies to patients.
Emerging Research and Developments
Emerging research in positron cancer treatment primarily focuses on refining therapeutic protocols and enhancing imaging capabilities. One key area of study involves the development of novel radiopharmaceuticals that can improve tumor targeting and reduce collateral damage to surrounding healthy tissues. For example, research is underway to identify new isotopes that emit positrons with optimal energy levels, improving resolution in imaging and efficacy in tumor destruction.
Additionally, studies are exploring how to combine positron emission tomography (PET) with other imaging modalities, such as magnetic resonance imaging (MRI) or advanced computed tomography (CT)**. This multimodal approach can provide richer data, allowing professionals to better understand tumor behavior and respond to treatment more effectively.
"Innovations in radiopharmaceuticals and imaging techniques can redefine our understanding and treatment of cancer."
Another promising direction involves the use of artificial intelligence and machine learning algorithms in interpreting PET scans. By analyzing patterns and anomalies in imaging data, these technologies can assist in making more personalized treatment decisions, predicting responses to therapy, and identifying potential metastases early.
The Role of Personalized Medicine
The concept of personalized medicine is increasingly relevant in the context of positron cancer treatment. By tailoring therapies to the individual characteristics of each patient and their specific type of cancer, healthcare providers can optimize treatment plans. This approach minimizes unnecessary side effects and enhances treatment outcomes.
Personalized medicine relies heavily on molecular profiling of tumors. By analyzing biomarkers associated with cancer progression, clinicians can select the most suitable positron-based therapies that are specifically effective for a patient's unique tumor biology. For instance, targeting specific pathways that are active in a patient’s tumor can lead to more successful outcomes with fewer adverse effects.
Moreover, integrating patient health data with treatment plans ensures that factors such as genetic makeup, prior treatments, and overall health are considered. This complexity highlights the need for ongoing collaboration between oncologists, radiologists, and researchers to develop sophisticated models that can predict how a patient might respond to positron treatment modalities.
Ending
Recapping Key Insights
The exploration of positron cancer treatment has unveiled numerous insights that enrich the understanding of its application in clinical settings.
- Precision in Diagnosis and Treatment: Positron emission tomography (PET) provides greater accuracy in identifying tumors, enabling better treatment planning.
- Advancements in Radiopharmaceuticals: The development of specialized radiopharmaceuticals enhances targeted therapy options, improving patient outcomes significantly.
- Integration of Technologies: The merging of PET with MRI and CT scans adds layers of information, allowing for comprehensive diagnosis and monitoring.
- Challenges and Solutions: Current limitations, such as accessibility and specific patient factors, highlight the need for innovative solutions in treatment protocols.
These points underscore the progress made and the potential for future enhancements within the realm of positron cancer treatment.
Final Thoughts on Advancements in Treatment
The therapy's ability to be tailored to individual patient needs not only improves efficacy but also minimizes adverse effects. Given the growing body of evidence supporting the efficacy of positron-based methods, ongoing investment in research will likely yield further innovations.