Research on Solar Cells: Trends and Future Directions
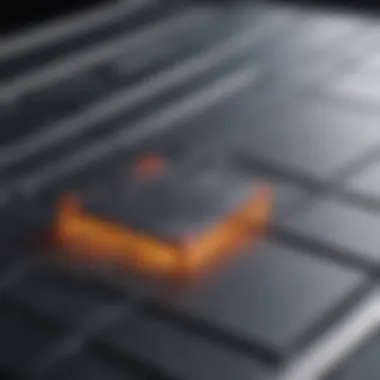
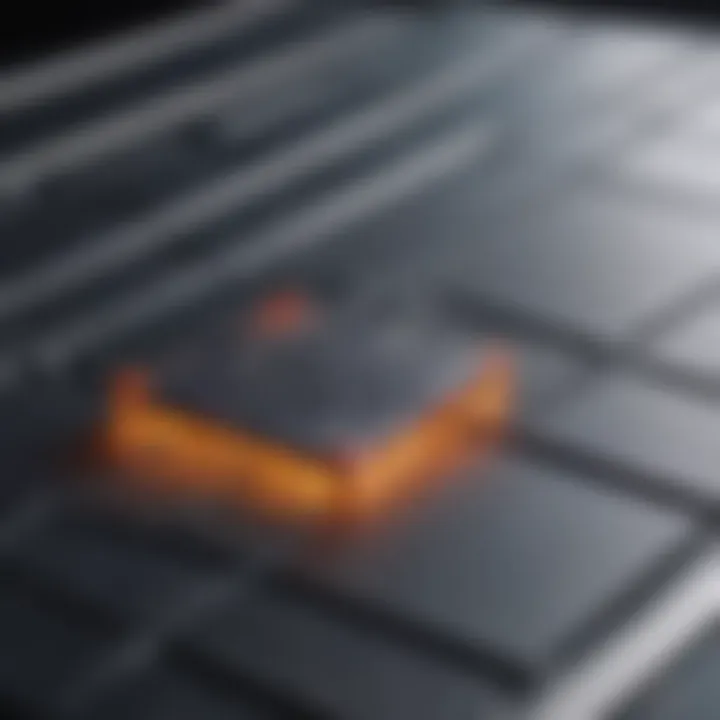
Intro
Research in solar cell technology has seen significant evolution in recent years. The push towards cleaner energy sources has made solar power an essential area of study. Engaging with advancements in solar cells is crucial for harnessing solar energy effectively. This article aims to unpack the ongoing trends, breakthroughs, and future directions in solar cell research.
Research Overview
Summary of Key Findings
The examination of current studies reveals varied improvements in solar cell efficiency. Notable findings include the development of perovskite solar cells that offer higher efficiency rates compared to traditional silicon cells. Studies indicate that combining different solar cell technologies can yield enhanced performance. Furthermore, the introduction of advanced materials has shown a potential to lower production costs while improving energy conversion.
Background and Context
Solar energy has emerged as a pivotal component in the global energy transition. Countries worldwide are investing in solar technologies to reduce reliance on fossil fuels. Historical reliance on silicon-based solar cells dominated the market for years. However, recent advancements suggest a shift towards new materials, such as perovskites and organic photovoltaics. These innovations not only promise better efficiency but also the potential for flexible and lightweight applications.
Methodology
Experimental Design
To understand the trends effectively, various experimental designs have been employed across studies. These often include comparative analyses between traditional and emerging solar cells. Researchers focus on determining efficiency, stability, and cost-effectiveness through controlled experiments.
Data Collection Techniques
Common methods for data collection involve laboratory testing and field studies. Laboratory tests typically measure solar cell performance under controlled light conditions. Field studies evaluate how solar cells perform in real-world environments. Data gathered helps researchers assess the practicality of solar technologies.
"The continual progress in materials and structures is likely to redefine solar energy's role in global energy production."
The exploration of solar cell technologies not only opens new avenues for energy sources but also emphasizes the need for sustainable practices. With ongoing investments and research, the future directions of solar cell development will likely lead to significant advancements that are critical for global energy needs.
Prelude to Solar Cells
The realm of solar energy conversion has rapidly evolved, carving a significant niche in the global energy landscape. Solar cells hold the potential to transform how we harness energy from the sun. They enable not only power generation but also contribute to sustainability and reduction of our carbon footprint. The examination of solar cells is critical as their deployment can mitigate climate change effects and advance energy independence.
Understanding the foundational aspects of solar cells provides insights into their functionality and historical development, which are essential for discussing current trends and future directions in this field.
Definition and Functionality
Solar cells, also known as photovoltaic (PV) cells, are devices that convert light energy directly into electrical energy through the photovoltaic effect. This phenomenon occurs when photons from sunlight strike the cell surface, exciting electrons and generating a flow of electric current. The basic structure typically consists of semiconductor materials such as silicon, which plays a crucial role in this conversion process.
The primary functionality of solar cells resides in their ability to capture sunlight and convert it into usable energy. This energy can be utilized in various forms, from powering homes to feeding into the electrical grid. Moreover, solar cells are integral to reducing dependence on fossil fuels, thus making them vital in the discourse on renewable energy sources.
Historical Development
The journey of solar cells began in the 19th century with the discovery of the photovoltaic effect, but practical applications started to gain traction post-1950s. Early solar cells were expensive and primarily used in space applications. Over time, advancements in materials and technology have significantly reduced costs and improved efficiency.
By the 1970s, mass production techniques allowed for wider availability, fostering growth in residential and commercial applications. The inceptive role of government incentives and environmental consciousness has propelled this momentum, leading to the installation of solar arrays on rooftops and large-scale solar farms today.
Recent years have demonstrated an acceleration in research around solar cell efficiency and material innovation, which hints at a promising future for solar technology. As public interest and investment flow towards sustainable energy, the solar cell landscape is set for continued transformation.
Types of Solar Cells
The landscape of solar energy is diverse, as it comprises various types of solar cells, each with distinct characteristics and advantages. Understanding these differences is crucial for both researchers and practitioners in the field. The type of solar cell impacts not only the efficiency of energy conversion but also the cost, installation requirements, and suitability for specific applications. This section delves into the main types of solar cells, providing insight into their respective benefits and considerations.
Monocrystalline Silicon Cells
Monocrystalline silicon cells are known for their high efficiency and longevity. These cells are made from a single continuous crystal structure, which results in a uniform appearance and high purity. The efficiency rates for monocrystalline cells typically range from 15% to over 22%. This is due to their ability to convert sunlight into electricity more effectively than many other types. Furthermore, their long lifespan of over 25 years makes them a reliable option for many installations.
Advantages of monocrystalline cells include:
- Higher efficiency compared to other solar cell types.
- Space-efficient, as they produce more power per square meter.
- Well-established technology with a proven track record.
However, they are generally more expensive to produce, which may deter some users. Additionally, their performance can degrade slightly in high temperatures, which is an important factor to consider in certain climates.
Polycrystalline Silicon Cells
Polycrystalline silicon cells, unlike their monocrystalline counterparts, are composed of multiple silicon crystals melted together. This structure makes them easier and less expensive to manufacture. The efficiency of polycrystalline cells is typically about 13% to 16%, which is slightly lower than that of monocrystalline cells.
Their benefits include:
- Cost-effective production, leading to lower prices for consumers.
- Less energy-intensive manufacturing process.
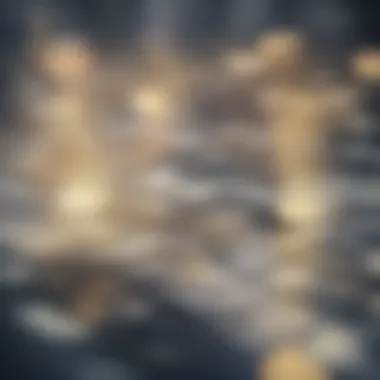
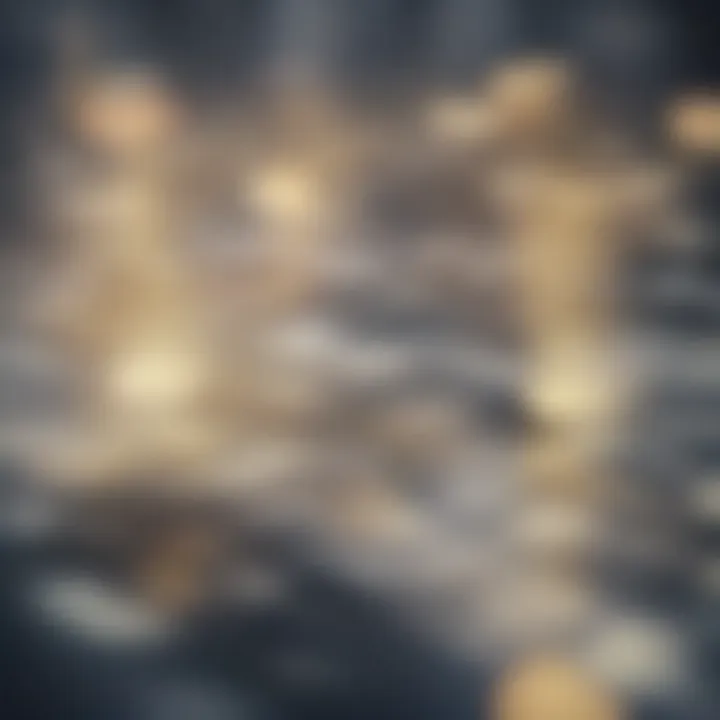
On the other hand, polycrystalline cells tend to occupy more space to generate the same amount of power as monocrystalline cells. Their performance can also be less efficient in limited sunlight conditions. This can be a consideration in regions that experience such conditions frequently.
Thin-Film Solar Cells
Thin-film solar cells are a different approach entirely, utilizing layers of photovoltaic material that are only a few micrometers thick. These cells can be made from materials like cadmium telluride or amorphous silicon. A significant advantage is their flexibility, allowing integration into various surfaces, such as building materials and vehicles.
Key aspects of thin-film solar cells include:
- Lower manufacturing costs.
- Lightweight and flexible, enabling innovative applications.
- Better performance in low-light conditions.
However, they generally have lower efficiency rates, around 10% to 12%. The area required for installation may be larger compared to crystalline options. Thus, while they offer unique opportunities, their usage often depends on the specific application and space constraints.
Emerging Technologies
Emerging technologies in solar cell development are pushing the boundaries of efficiency and application. Organic photovoltaic (OPV) cells, perovskite solar cells, and tandem cells are examples of innovations currently in research and early-stage commercialization.
- Organic photovoltaic (OPV) cells: These cells use organic materials and offer flexibility and low weight. Their development is promising but still in the early phases.
- Perovskite solar cells: Renowned for their rapid advancements in efficiency, perovskite cells have reached over 25% in lab settings. Their versatility and lower manufacturing costs reflect a significant shift in solar technology.
- Tandem cells: These involve stacking several layers of different materials to capture more sunlight spectrum, effectively increasing efficiency.
These emerging technologies hold potential for improved economics and efficiency, but challenges such as stability and large-scale manufacturing remain.
Efficiency and Performance
Efficiency and performance are core to the development and adoption of solar cell technologies. As the world increasingly shifts towards renewable energy sources, understanding how to maximize the output of solar cells becomes essential. The performance of solar cells directly impacts their viability as an energy source, influencing economic considerations and environmental sustainability. This section aims to delve into the different facets of measuring solar cell efficiency, the factors that influence it, and recent advancements that seek to improve this crucial aspect of solar technology.
Measuring Solar Cell Efficiency
The efficiency of solar cells is defined as the percentage of sunlight that can be converted into usable electricity. To measure this efficiency, various methodologies are employed, including standardized test conditions where light intensity, temperature, and atmospheric conditions are controlled. Performance metrics can vary based on the type of solar cell, with monocrystalline cells often showing higher efficiencies compared to other types.
Common metrics that researchers focus on include:
- Power Conversion Efficiency (PCE): This is the primary indicator of a solar cell's effectiveness.
- Fill Factor (FF): This represents the quality of the solar cell and affects its maximum power output.
- Open-circuit Voltage (Voc) and Short-circuit Current (Isc): These parameters help assess the electrical performance of the cell.
The importance of accurate measurements cannot be overstated. They provide a benchmark for comparing different technologies and guide further research efforts.
Factors Influencing Efficiency
Multiple elements influence the efficiency of solar cells. Some of the main factors include:
- Materials Used: The choice of material can determine how much light a solar cell can absorb and convert into electricity. For instance, perovskite materials have sparked interest for their potential to exceed traditional silicon efficiencies.
- Environmental Conditions: Factors such as shading, temperature, and pollution can significantly affect performance. Higher ambient temperatures often reduce efficiency.
- Construction Quality: Manufacturing defects or variations in material properties can lead to inefficiencies. Ensuring high-quality production processes is critical.
- Angle of Installation: Solar panels should be optimally positioned to capture maximum sunlight, depending on geographic location.
Understanding these factors is vital for both manufacturers and researchers aiming to create more efficient solar technologies.
Recent Advancements in Efficiency
Innovation drives continued improvements in solar cell efficiency. Recent advancements include:
- Tandem Solar Cells: This technology combines two different materials, allowing more spectral coverage of sunlight, capturing more energy.
- Bifacial Solar Cells: These cells can collect sunlight from both sides, which increases their overall efficiency, especially in reflective environments.
- Light Management Techniques: Nano-coating and textured surfaces have been developed to improve light absorption and reduce reflection losses.
- Advanced Manufacturing Techniques: Improved material processing methods are aiding in the development of high-efficiency solar cells.
These advancements illustrate a trend towards not only enhancing performance but also addressing the pressing challenges of energy demands globally. Each innovation offers new potential and signifies the ongoing quest for optimal solar energy solutions.
"The future of solar technology lies in harnessing new materials and innovative designs to push the boundaries of efficiency."
Materials in Solar Cell Research
In the rapidly evolving landscape of solar technology, the materials used in solar cells play a crucial role. Understanding these materials is essential for improving efficiency, reducing costs, and enhancing the sustainability of solar energy systems. Each type of material comes with its unique advantages and challenges, influencing the performance, lifespan, and manufacturing processes of solar cells. As researchers strive for innovations, the choice of materials becomes a focal point.
Conventional Materials
Conventional materials for solar cells primarily include silicon, which dominates the market due to its prevalence and established manufacturing processes. Silicon solar cells are known for their durability and efficiency, particularly in monocrystalline and polycrystalline forms. Monocrystalline silicon cells generally offer higher efficiency rates but can be more expensive to produce.
Key points about conventional materials include:
- Established technology with a proven track record.
- High efficiency levels, especially in favorable conditions.
- Significant investment in manufacturing infrastructure, which leads to economies of scale.
However, conventional solar technologies face limitations. For instance, silicon is energy-intensive to produce. Moreover, its performance can be affected by temperature fluctuations and shading. These challenges drive research into alternative materials that may offer better performance at lower costs.
Perovskite Materials
Perovskite materials have emerged as a promising alternative to traditional solar cell materials. Their unique crystal structure allows for high absorption efficiency and the potential for lower production costs. This can be especially significant in making solar technology more accessible.
Noteworthy points about perovskite materials include:
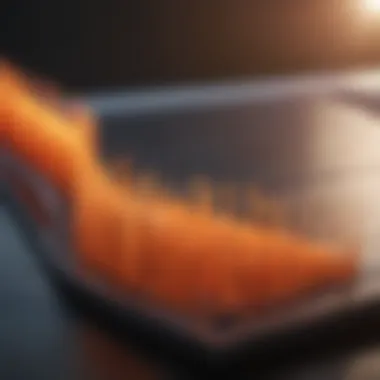
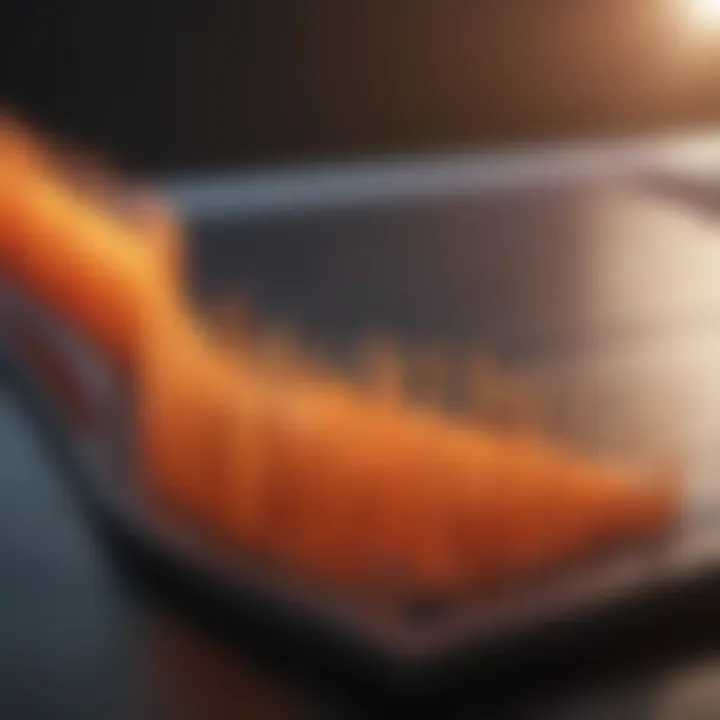
- High absorption efficiency leading to competitive power conversion rates.
- Simplicity in manufacturing processes, which can lower costs.
- Flexibility in application, allowing integration with existing solar technologies.
Despite these advantages, perovskite materials face challenges, particularly regarding stability and scalability. Researchers are actively looking into these issues, as enhancing durability and overcoming production challenges will be key to realizing their potential. Some studies indicate that incorporating novel additives or combining perovskites with other materials could mitigate these concerns.
Nanomaterials and Their Applications
The exploration of nanomaterials in solar cell research is expanding due to their unique properties that can enhance efficiency and reduce costs. Nanostructured materials, such as quantum dots and nanocrystalline films, open new avenues for solar cell design and function.
Benefits of incorporating nanomaterials include:
- Enhanced light absorption, increasing the cellโs efficiency.
- The potential for lightweight and flexible solar panels, expanding installation capabilities.
- Tailored electronic properties by modifying size and surface characteristics.
Although the field holds great promise, there are challenges yet to overcome. The scalability of nanomaterials is still under investigation, and their long-term performance is not fully understood. Addressing these issues is essential for future advancements in solar technology.
Environmental Impact and Sustainability
Understanding the environmental impact and sustainability of solar cell technology is vital in the context of global energy transformations. As nations strive to reduce greenhouse gas emissions and lessen reliance on fossil fuels, solar energy emerges as a compelling alternative. However, its production and end-of-life management must be carefully evaluated to ensure that it contributes positively to environmental goals rather than introducing new problems. This section examines key elements related to the lifecycle of solar cells, their sustainability practices, and the implications for various stakeholders.
Lifecycle Analysis of Solar Cells
Lifecycle analysis (LCA) of solar cells provides an in-depth view of their environmental effects from production to disposal. The process breaks down each phase: raw material extraction, manufacturing, distribution, usage, and end-of-life.
During raw material extraction, the environmental impact varies based on which resources are used. For instance, silicon, a primary component in many solar cells, is derived mainly from quartz, which requires intensive mining practices. Manufacturing processes also consume significant energy, potentially sourcing from non-renewable origins. A critical part of LCA is quantifying the carbon footprint associated with these steps.
The operational phase is often where solar cells shine, emitting little to no carbon emissions during their energy generation lifespan. Studies suggest that solar panels offer a net positive environmental impact after just a few years of operation.
Conversely, the end-of-life phase poses challenges. Disposing of solar cells can lead to environmental hazards if not managed correctly. Toxic materials often found in some panels, like cadmium and lead, may leach into the environment if not processed appropriately.
It is crucial to implement incentives for manufacturers and consumers to participate in recycling programs and to develop standards that promote responsible disposal methods.
Recycling and End-of-Life Considerations
Recycling is an essential aspect of the sustainability of solar cells. As the market expands, the volume of solar panels reaching their operational limit is increasing. Proper recycling of solar panels presents multiple benefits, including material recovery and reduction of environmental hazards.
Currently, only a fraction of solar panels are recycled at their end of life. Innovative technologies, such as the use of hydrometallurgical processes, have shown promise in reclaiming valuable materials from old panels. These processes not only minimize waste but also reduce the mining pressure on virgin materials.
"To ensure that solar energy remains a sustainable choice, systemic recycling protocols and facilities must be established."
Governments, researchers, and companies are collaborating in developing frameworks to facilitate the recycling of solar modules. This includes standards that encourage manufacturers to design for recyclability from the start. Through such initiatives, stakeholders benefit from a circular economy, conserving resources, and reducing overall environmental impacts.
Economic Considerations
Economic factors play a crucial role in the development and adoption of solar cell technology. These considerations encompass cost trends, market dynamics, and the global adoption rates of solar solutions. Understanding the economics of solar cells is essential not only for researchers and industry stakeholders but also for policymakers and consumers looking to make informed decisions. With the increasing urgency to address climate change, efficient allocation of resources is paramount. Economic considerations directly impact the growth trajectory of solar technology, shaping it into a feasible alternative to traditional energy sources.
Cost Trends in Solar Technology
The reduction of costs in solar technology has been a significant driver in its growth. Over the past decade, the price of photovoltaic (PV) cells has decreased dramatically due to advancements in manufacturing processes and economies of scale. For instance, the average cost to install solar panels has dropped by more than 80% since 2010. This makes solar energy increasingly accessible for both residential and commercial users.
Moreover, various factors influence these trends:
- Technological advancements: Innovations in production efficiency lead to lower material costs.
- Increased competition: Entry of new manufacturers fosters price reductions and better service offerings.
- Government incentives: Subsidies and tax benefits for solar installation encourage wider adoption, helping offset initial investments.
This reduction in costs has resulted in solar energy becoming competitive with fossil fuels, shifting the energy landscape significantly.
Market Dynamics and Global Adoption Rates
The dynamics of the solar market are complex and influenced by several factors including regulation, technology, and consumer preferences. The International Energy Agency (IEA) indicates that solar power capacity has surged, positioning it as one of the fastest-growing energy sources globally.
Key elements impacting these dynamics include:
- Policy frameworks: Many governments are implementing policies that facilitate investment in renewable energies. Net metering and feed-in tariffs are examples that promote solar adoption.
- Consumer awareness: Increased public knowledge around the environmental benefits and long-term savings associated with solar technologies have led to heightened demand.
- Global trends: Countries like China and the United States are leading the charge, but adoption rates vary by region due to factors such as available sunlight and infrastructure development.
Overall, the global market for solar technology is growing rapidly, evidenced by the increasing number of installations and investments into research and development.
Solar energy is more than just a sustainable option; it is becoming a viable economic choice for individuals and businesses alike.
Current Research Trends
Current research in solar cell technology plays a pivotal role in enhancing the efficiency and effectiveness of solar energy as a renewable resource. This section aims to detail the innovative approaches taken to improve output, as well as the interdisciplinary contributions that enrich the field. By understanding these trends, stakeholders can better appreciate the developments that are shaping the future of solar technology and its integration into global energy systems.
Innovative Approaches to Increase Output
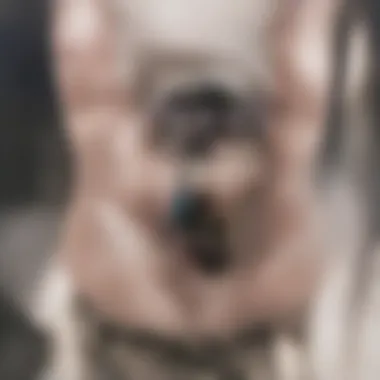
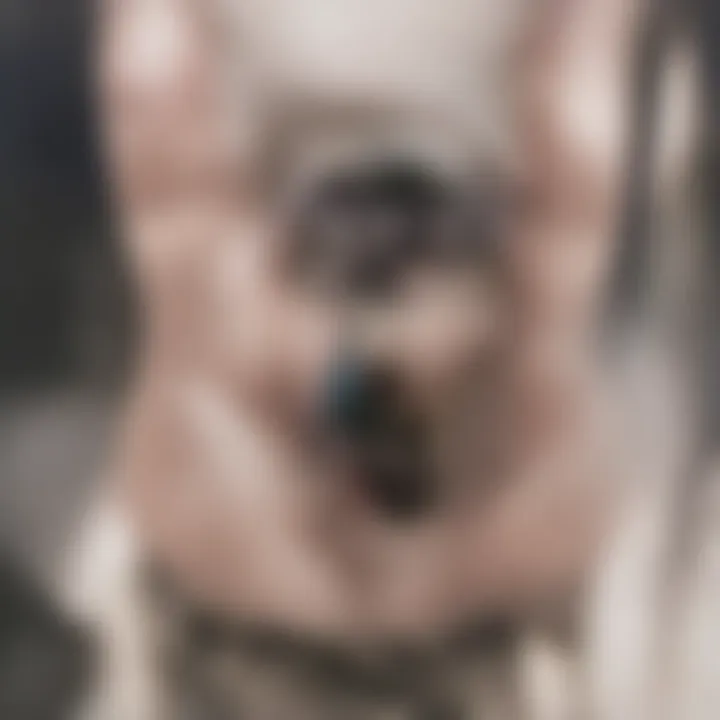
One noticeable trend in solar cell research is the exploration of innovative methods to boost output. Researchers are constantly pushing the boundaries of technology to enhance the performance of solar cells. Different techniques are being developed and tested.
- Bifacial Solar Cells: These cells can absorb light from both sides, maximizing the amount of solar energy captured. This setup has shown significant efficiencies in suitable environments.
- Tandem Solar Cells: Combining two different types of solar cells can capture a broader spectrum of light. For instance, pairing perovskite solar cells with silicon cells leads to higher efficiencies compared to standard solar technology.
- Light Management Techniques: Utilizing textures or coatings on solar cells can reduce reflection and enhance light absorption, contributing to better energy conversion rates.
- Advanced Manufacturing Methods: Techniques such as 3D printing and laser scribing are becoming popular for creating more precise and efficient solar cells, achieving higher production yields at reduced costs.
The implementation of these innovative approaches indicates that the field is dynamic, offering real promise for increasing the viability of solar energy.
Interdisciplinary Research Contributions
The field of solar energy does not exist in isolation. It intersects with numerous disciplines, enhancing the research outcomes. Some notable interdisciplinary avenues include:
- Materials Science: This field contributes advancements in new materials, specifically perovskites, which have emerged as a strong competitor to traditional silicon. Progress here impacts efficiency on a fundamental level.
- Engineering: Various engineering disciplines, including electrical and mechanical engineering, collaborate to devise better photovoltaic systems that can withstand environmental stressors while maintaining high performance.
- Environmental Science: Understanding the ecological impacts of solar panel production, use, and disposal is vital for sustainability. This awareness pushes the boundaries of design to ensure minimal negative consequences on the environment.
- Economics: The market dynamics of solar energy production and consumption are influenced by economic research, helping to address cost efficiency and strategize broader adoption.
Innovative solutions in solar technology often emerge from these collaborative efforts, resulting in a more robust and effective approach to renewable energy.
In summary, current research trends in solar cell technology emphasize the importance of innovative output-enhancing approaches and interdisciplinary collaboration, which are crucial for advancing solar energy's role in sustainable development.
Challenges in Solar Cell Technology
Challenges in solar cell technology represent significant hurdles that must be navigated to advance solar energy as a viable solution for global energy demands. These challenges not only impede the efficiency of solar cells but also affect the market adoption and integration of solar technologies into existing energy systems. Recognizing and addressing these obstacles is crucial for researchers, manufacturers, policymakers, and environmental advocates who aim to enhance renewable energy's role in combating climate change.
Technical Obstacles
Technical obstacles in solar cell technology primarily revolve around efficiency and performance limitations. One major issue is the physical constraints imposed by materials used in solar cells. For instance, traditional silicon cells have a maximum theoretical efficiency limit, known as the Shockley-Queisser limit. While recent innovations, such as tandem solar cells made from perovskite and silicon, attempt to break through these limits, they introduce new challenges related to stability and degradation.
Furthermore, the production processes for solar cells often lead to defects that compromise overall efficiency. For example, impurities during the manufacturing of silicon wafers can significantly reduce the effectiveness of the cells. The complexity of scaling up production while maintaining quality adds another layer of difficulty. Researchers continuously explore alternative materials, like copper indium gallium selenide, which may improve efficiency but also bring their own sets of challenges in the production and application.
Regulatory and Market Barriers
Regulatory and market barriers present another significant challenge for solar technology. The solar industry is heavily influenced by governmental policies, incentives, and regulations. Inconsistent policies across different regions can create uncertainty for investors and developers, leading to reduced investment in solar technologies.
Additionally, integration into existing energy grids can be problematic. Many countries lack the infrastructure to support large-scale solar energy generation, which requires careful planning and investment for grid upgrades. Market dynamics also play a role; for instance, fossil fuel subsidies can distort market conditions and undermine the competitiveness of solar energy.
The end-users, including residential and commercial consumers, often face additional barriers. Initial installation costs, despite decreasing over time, can still deter adoption, and the perceived reliability of solar energy can be a concern. Understanding that these regulatory and market barriers exist is critical for stakeholders who aim to navigate the evolving landscape of solar energy.
"Overcoming both technical and regulatory challenges is essential to harnessing solar energy's full potential as a clean energy source."
Addressing these challenges requires coordinated efforts between researchers, industry, and government bodies. Solutions might include increased funding for research and development, incentives for clean energy investments, and a better regulatory framework that supports innovation and market entry. Ultimately, addressing the challenges in solar cell technology is not just crucial for the advancement of solar energy but is vital for the transition to a sustainable future.
Future Directions in Solar Cell Research
The pursuit for advanced solar cell technology is crucial for various reasons. First, with the global push towards sustainable energy solutions, understanding future directions in solar energy adoption is very essential. It enables researchers, educators, and industry professionals to align their efforts with trends that can significantly affect energy systems worldwide. Emphasizing future advancements could lead to improvements in efficiency, cost reduction, and accessibility, all of which are key components for widespread solar adoption.
Predictions for Upcoming Innovations
Several emerging areas indicate where solar cell technology might head in the near future.
- Perovskite Solar Cells: These cells are gaining much interest due to their potential for high efficiency combined with lower production costs. Research is ongoing to enhance the stability and longevity of these cells.
- Bifacial Solar Panels: Utilizing both sides of the panel to capture sunlight is another innovation that could increase energy yield significantly.
- Building-Integrated Photovoltaics (BIPV): The integration of solar cells into building materials could change the landscape of urban energy usage, making solar power more ubiquitous and convenient.
- Flexible Solar Cells: There is a growing interest in developing lightweight, flexible solar technology that can be applied to various surfaces, increasing installation versatility.
- Enhanced Energy Storage Solutions: Innovations in battery storage, alongside solar technology, are essential to overcome the intermittent nature of solar energy.
Each of these innovations presents not only technical challenges but also opportunities for substantial advancements in solar efficiency and versatility.
Role of Solar Energy in Future Energy Systems
The integration of solar energy into future energy systems is crucial to achieving sustainability goals. Increasing reliance on renewable energy sources is a paramount step in reducing greenhouse gas emissions. Solar energy, due to its scalability, versatility, and declining costs, is expected to play a core role in the global energy infrastructure.
- Decentralized Energy Production: Solar allows for localized energy generation, thus reducing transmission losses and improving grid stability.
- Grid Modernization: Solar energy can be an integral part of the smart grid framework, where energy production and consumption can be managed more efficiently.
- Collaboration with Other Technologies: Integrating solar power with other renewable sources, such as wind and hydro, can provide a more stable energy supply.
"To maximize solar energy's potential, advancements must align with policy frameworks and market dynamics to fully realize its benefits."
By anticipating these developments and their potential impact, stakeholders can better navigate the challenges associated with the transition to solar energy.
The End
The conclusion serves as a vital part of this article by encapsulating the findings and perspectives provided throughout the sections. It acts as a framework that ties together the various strands of research on solar cells and their evolving technologies. By highlighting the critical insights, the conclusion sheds light on how advancements are shaping the future of renewable energy.
Summation of Key Insights
In summary, the research discussed in the preceding sections reveals that solar cells have transitioned significantly in their development. Current technologies have achieved remarkable efficiency levels due to innovations in materials and design. For instance, the exploration of perovskite materials has opened new avenues for higher conversion rates. Additionally, challenges such as technical limitations and market barriers have been thoroughly examined, emphasizing the need for ongoing research and collaboration among stakeholders. Key insights include:
- Innovations in monocrystalline and polycrystalline technologies continue to dominate,
- Emerging thin-film technologies are promising for flexible applications,
- Interdisciplinary efforts are essential in overcoming hurdles and driving adoption,
- Environmental considerations in lifecycle assessments remain a critical factor.
Implications for Stakeholders
For stakeholders involved in solar energy, including investors, manufacturers, and policymakers, the implications of the findings are significant. They must adapt to the fast-changing landscape of solar technology by investing in research and development, focusing on sustainable practices, and staying informed about regulatory changes. Stakeholders should also prioritize:
- Collaboration with research institutions to promote innovative solutions,
- Engaging with communities to raise awareness of solar benefits,
- Investing in education and training programs to grow the workforce,
- Exploring financial models that can foster the adoption of clean energy solutions.
Understanding these implications will enhance strategic planning and decision-making in the realm of solar cells. Overall, it is clear that solar energy plays a vital role in alternative energy systems, contributing to a sustainable future.